This topic is essentially a continuation of topic 6 and 11, building on previous knowledge while introducing a few new concepts. The topic continues with explaining the internal organs by introducing the last major abdominal organ (the liver) and explaining some other known organs with further detail. I found the topic very comprehensible since the book has already built a solid foundation on most of the organs, so it should not be a very challenging or eye-opening chapter to read trough.
Points for revision:
- Essential nutrients and dietary conditions
- Exocrine secretions in digestion
- Other properties of the liver
- The pituitary gland and the hypothalamus
- Hemoglobin and oxygen affinity
- Oxygen dissociation curves and the Bohr shift
- Dissociation curves of myoglobin and fetal hemoglobin
Essential nutrients and dietary conditions
Nutrients can be divided into 2 groups, the essential and the unessential. Essential nutrients are nutrients which the body cannot synthesize, so must therefore be consumed through the diet. Among the essential nutrients are most minerals, vitamins, a few amino acids, and a few fatty acids.
Minerals such as iron and calcium are important because they make up structures in our bodies such as hemoglobin and the skeleton. Furthermore, a lot of minerals act as electrolytes in our bodies since they carry charges. Many of the minerals such as sodium, potassium, and calcium and their functions in for example action potentials should already be familiar. Minerals are usually long lived in our bodies since they can be recycled, however, since the recycling is not 100% efficient, our bodies require an intake of minerals from time to time. Deficiencies in minerals such as calcium during growth can cause rickets, which is a bone malfunction due to the body not having enough material available, and deficiencies in iron can cause low hemoglobin levels which can lead to for example fatigue. Women generally require a higher intake of iron than men since iron is lost monthly during menstruation.
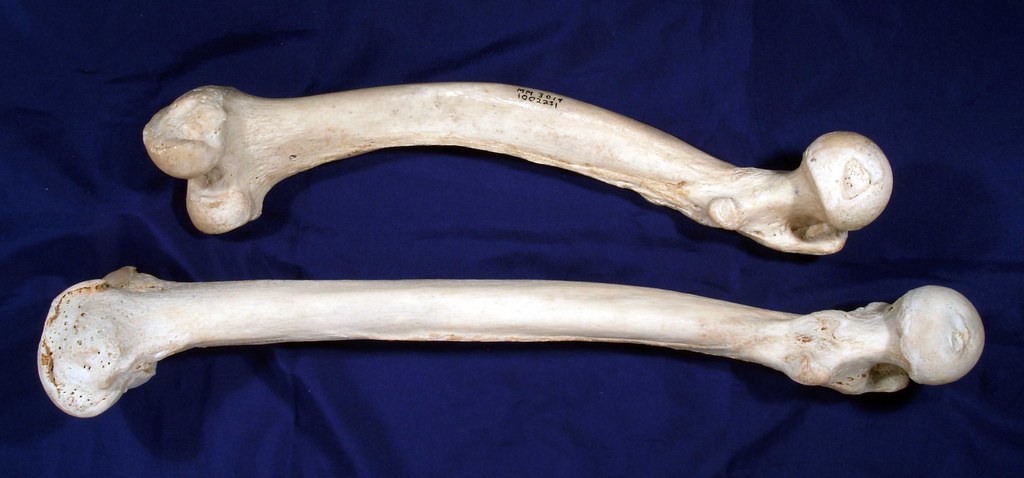
Vitamins are also important to us since they participate in many vital functions such as maintaining the immune system and growth. Since humans cannot synthesize most vitamins, they must be consumed through the diet and thus classified as essential. One exception is vitamin D, which the body can actually synthesize in the epidermis with the help of uv light from the sun. However, too much exposure to the sun can cause other health issues such as skin cancer, so the exposure should be balances. It is recommended to get at least 30 minutes of sunlight each day. Other sources of vitamin D are for example fatty fish and meats. Since vitamin D is important for the growth of the skeleton, deficiencies in vitamin D can also cause rickets and other skeleton conditions such as osteomalacia. Osteomalacia is a softening of bones due to the lack of vitamin D or calcium. Osteomalacia typically occurs in adults, since rickets is usually developed instead of osteomalacia during growth in children.
Another essential vitamin is vitamin C, which is probably the most well-known vitamin. Its fame can arguably be attributed to its importance in maintaining the immune system and the belief that it can protect against the flu. Even though the protection towards the flu is somewhat questionable, there is strong evidence that vitamin C does indeed play an important role in maintaining the immune system. Unlike vitamin D, our bodies cannot synthesize vitamin C, so it is classified as essential. A deficiency in vitamin C can cause scurvy which has symptoms such as swelling, bleeding, and pain. Scurvy was common amongst sailors due to the lack of fresh vegetables and fruits in their diet, which are major sources of vitamin C.
Among the 20 amino acids 9 of them are essential to us since we cannot synthesize or interconvert them. As amino acids are crucial in protein synthesis, it is important to have access to all of the amino acids in order to life functions. Since amino acids build up proteins, most protein rich foods such as meat and beans contain many essential nutrients and must therefore be incorporated into the diet. A condition associated with amino acids is phenylketonuria (PKU), which is an inability to metabolize the amino acid phenylalanine. The condition is a recessive autosomal condition and can therefore be inherited. Since there is no cure available for PKU, it has to be maintained by reducing the consumption of phenylalanine.
The last group of essential nutrients are the fatty acids, of which only 2 are essential and arguably the most well-known. Omega 3 and omega 6 differ chemically only in the positions of their double bonds and are therefore unsaturated fats. They are considered healthy fats because they are unsaturated and because they help regulate levels of cholesterol and blood pressure. An important source of omega 3 and omega 6 is fatty fish and fish oil. Many people take supplements in the form of fish oil, especially here in Norway during winter.
.jpg)
The above picture shows omega 3. Omega 6 is identical exept that the first double bond is located at the sixth carbon from the omega end. Omega 6 only have two double bonds.
Exocrine secretions in digestion
Exocrine glands secrete their contents into ducts which usually either leads to the surface of our bodies or the lumen of an internal organ. Examples of exocrine secretions to the surface are tears, saliva, and sweat, and secretions to the internal organs are gastric juice, pancreatic juice, and bile. All the mentioned internal secretions are important for digestion; however, we will firstly focus on gastric juice which is secreted in the stomach. The stomach is well-known for having a very acidic environment which is important for the activity of enzymes present in the stomach, and for the killing of harmful bacteria. The acidic environment is due to the secretion of hydrochloric acid (HCl) from glands in gastric pits. Gastric pits contain many different glands which all secrete their contents into the stomach to create gastric juice. The pits themselves are small “dips” into the lumen of the stomach which contain exocrine glands. Three important exocrine glands secrete hydrochloric acid, pepsinogen, and gastrin. Hydrochloric acid creates the acidic environment which the pepsinogen needs to become active, and gastrin creates a feedback loop which causes more secretion of hydrochloric acid and pepsinogen. Pepsinogen is the precursor to the protease pepsin, which becomes active once the hydrochloric acid converts pepsinogen into pepsin. The acidic environments also aid in killing harmful bacteria.
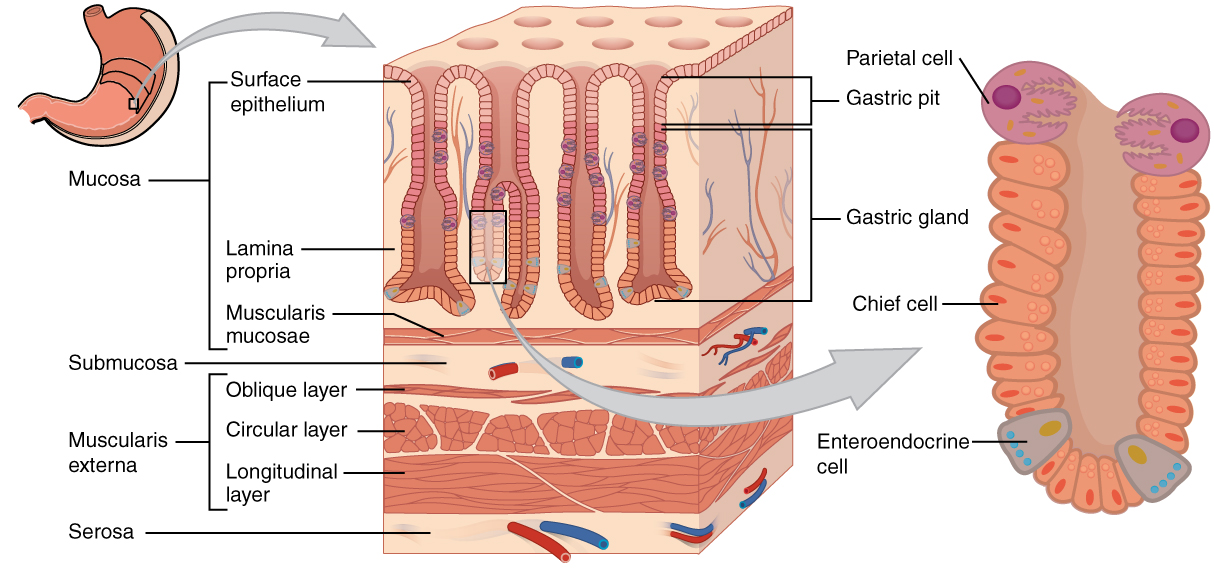
Intestinal villi
As previously explained, villi are hair-like projections in the small intestine that absorbs the digested nutrients in the lumen of the small intestine. Villi absorbs the nutrients by maximizing their surface area by adapting the hair-like structures in addition to incorporating microvilli which increases their surface area dramatically. Villi needs a large surface area because they absorb food by diffusion and active transport. However, the absorption needs to be selective since only the required and digested nutrients should be absorbed. Because the cells in the villi have an intercellular space between them, unwanted molecules can move between cells and enter the capillary bed. To avoid this, villi cells have membrane to membrane proteins that seals the intercellular gaps in what is called tight junctions. The tight junctions prevent undigested or unwanted molecules to be absorbed by the small intestine.
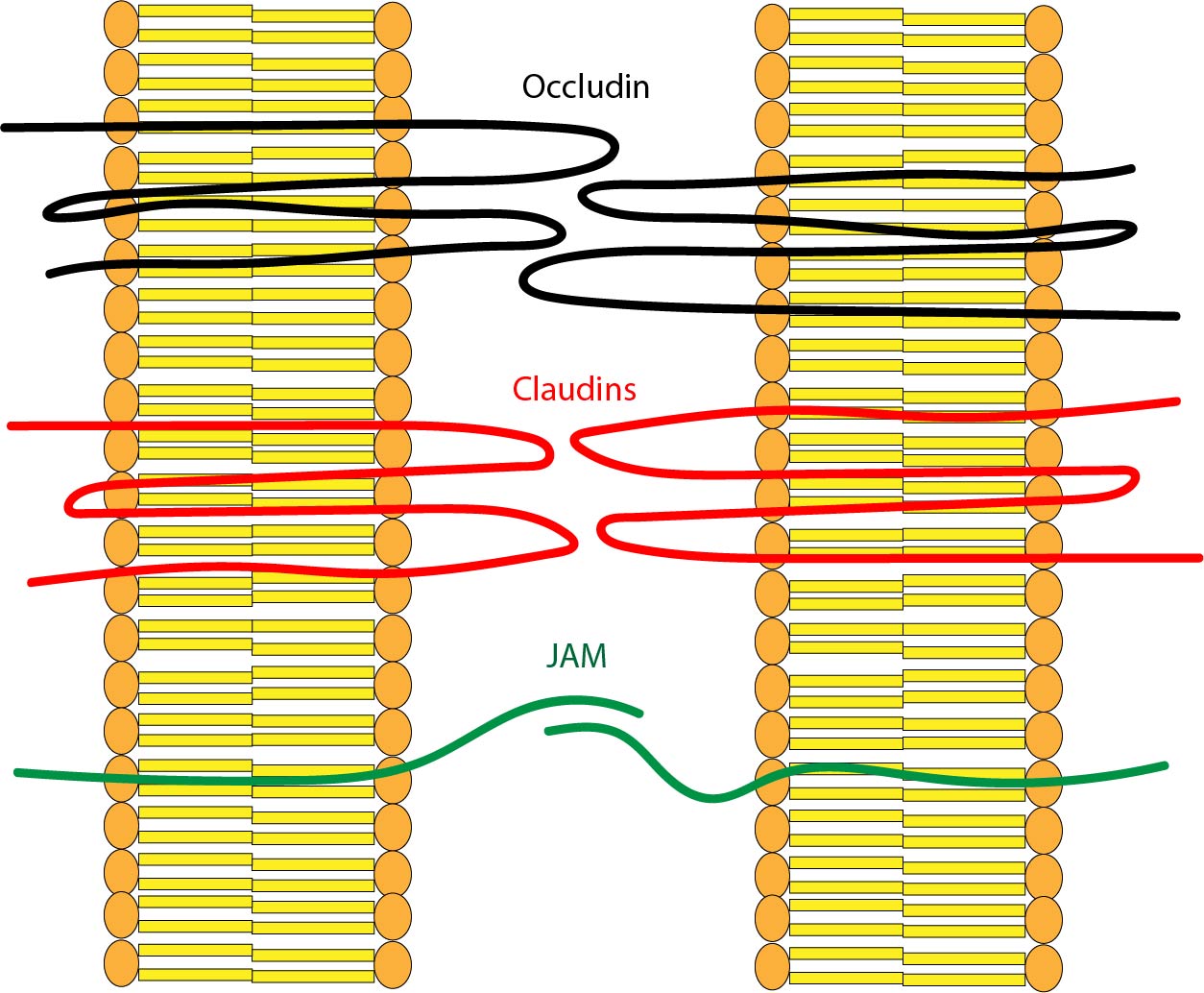
Anatomy of the liver
The anatomy of the liver is quite simple. Two blood vessels deliver blood to the liver, and one blood vessel outputs blood from the liver. The two blood vessels are the hepatic portal vein and the hepatic artery. The hepatic artery is what keeps the liver alive by delivering oxygenated and high-pressure blood directly from the heart. The hepatic portal vein is what delivers the contents that needs to be detoxified in the liver. The hepatic portal vein delivers low pressure deoxygenated and nutrient rich blood directly from the capillaries of the small intestine. This means that the liver is the first organ the digested nutrients enter in the body. The liver thus serves an important role in detoxing the body because the toxins reach the liver before it reaches the kidneys. The kidney itself is made of a specialized capillary bed called the sinusoid. The sinusoids are made up of liver cells called hepatocytes and cells called Kupffer cells. They both play an important role in detoxing. Another anatomical feature of the liver is the common hepatic duct. The duct is comprised of the right and left hepatic duct which combines into the common hepatic duct. The common hepatic duct transfers bile produced in the liver to the gall bladder which eventually releases it into the duodenum.
Detoxing in the liver
The removal of toxins by the liver happens in the sinusoids. The sinusoids are different from a normal capillary bed in that they are wider and contain two specialized cells, hepatocytes and Kupffer cells. Hepatocytes (liver cells) are in direct contact with the blood plasma as the sinusoid gets filled by blood. The hepatocytes can remove the toxins in the blood plasma by first chemically altering the toxins so that they become less toxic, and then altering them to make them water soluble. The more harmless toxins can now enter the kidneys to become part of urine, thus removing them from the body. The second type of cells in the liver, the Kupffer cells, are a type of phagocytic white blood cell that recycles hemoglobin and removes bacteria by phagocytosis. The lysosomes in the Kupffer cells then “digest” the contents and recycles them. Since hemoglobin is built up of 4 globin proteins and haem groups, the recycled components of hemoglobin are amino acids from the globin proteins and iron from the haem. The rest of the components are turned into bilirubin which is a key component of bile.
Other properties of the liver
Besides detoxing the blood, the liver has 2 other important functions. The first it the storage of nutrients, and the other is the production of bile and plasma proteins. As previously mentioned, the body maintains homeostatic glucose levels by regulating the glucose concentrations with two hormones called insulin and glucagon. When glucose levels are high, usually right after meals, the pancreas will start secreting insulin which will flow through the blood. The insulin will enter the sinusoids and stimulate the hepatocytes to increase the production of glycogen, which are large granules of glucose. This removes glucose from the blood and lowers blood glucose levels. The opposite action happens when blood glucose levels are too low. The pancreas then secretes glucagon, which stimulates the hepatocytes to convert glycogen into glucose again, thus restoring homeostasis.
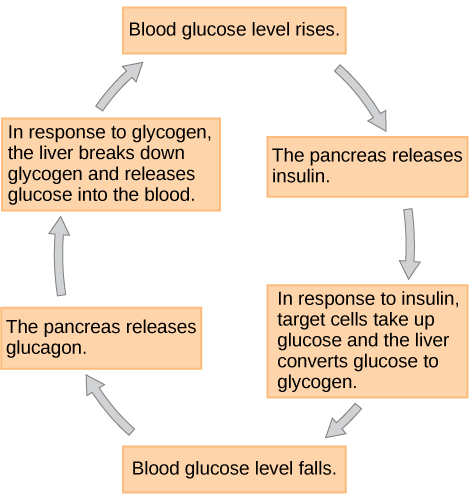
The other function of the liver is the production and secretion of bile and plasma proteins. Bile is primarily made of bilirubin which is produced by breaking down hemoglobin. Bile is important for digestion since it has emulsifying effects, which means that it “builds a bridge” between oil and water which makes them mix more throughout. This increases the surface area of the oil which makes it easier for lipase to break down fats. Another job of the liver is to produce plasma cells such as albumin and prothrombin. Albumin helps regulate osmotic blood pressure, while prothrombin is the first protein in the assembly line of blood clotting.
Cardiac muscle
Cardiac muscle is in many ways similar to skeletal muscle. However, cardiac muscles need to fulfill two criteria which skeletal muscle do not. Firstly, the muscle needs to contract quickly and uniformly. Secondly, it cannot tire since it needs to beat continuously. Cardiac muscle solves the first problem by joining the sarcomeres together with intercalated discs. These disks are joined together by gap junctions where the cytoplasm of one cell connects to the cytoplasm of another cell, in effect connecting all the cells together making one big unit. Additionally, cardiac muscles form branched structure which creates a “web” of muscle. These factors allow the muscle to contract quickly and uniformly.
Cardiac muscle solves the second problem by being rich in enlarged mitochondria which supplies a large power output to the heart in addition to being highly vascular, which means that it has a large blood supply. These two factors in combination allows the heart to work continuously without tiring, beating away for an entire lifespan.
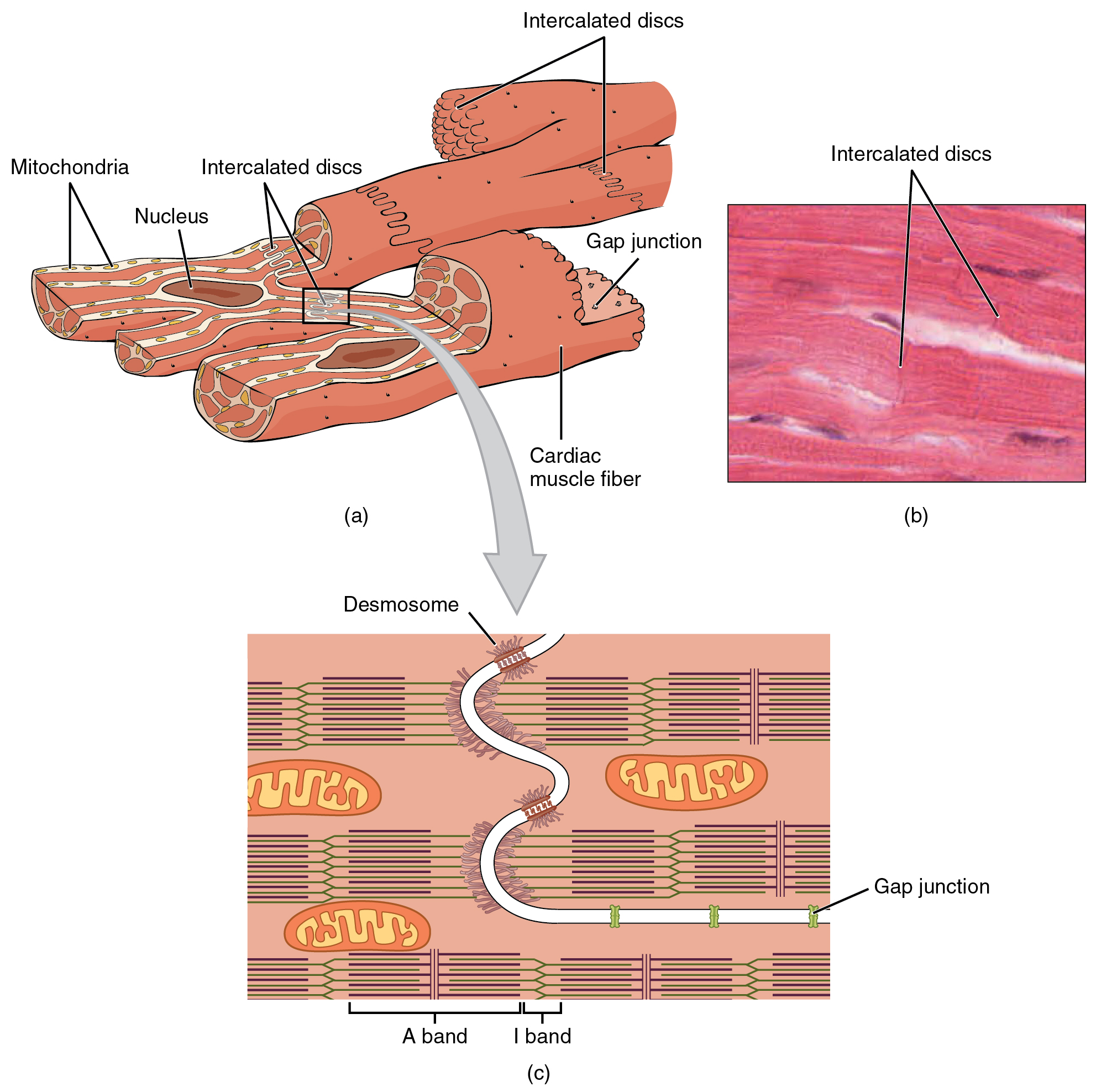
Cardiac cycle
During rest the heart can control its rhythm on its own due to its internal pacemaker called the sinoatrial node (SA node). The SA node is comprised of a specialized tissue capable of generating electrical impulses which cases both the atria to contract at the same time. When a heart chamber contracts it is said to be systole, and when it is not contracting it is said to be diastole. When the SA node sends a signal to contract, it sends a delayed signal to the atrioventricular node (AV node) which contracts both ventricles. The delayed signal is sent through fibers called Purkinje fibers. These fibers spread from the SA node through the ventricles which cases a unified systole. The cardiac cycle thus starts with the SA node contracting the atria, which pumps blood through the atrioventricular valves into the ventricles. The delayed signal from the SA node to the AV node then contracts the ventricles which pumps blood out of the semilunar valves and out of the heart. The two “lub” sounds of the heartbeat comes from the closing of the valves.
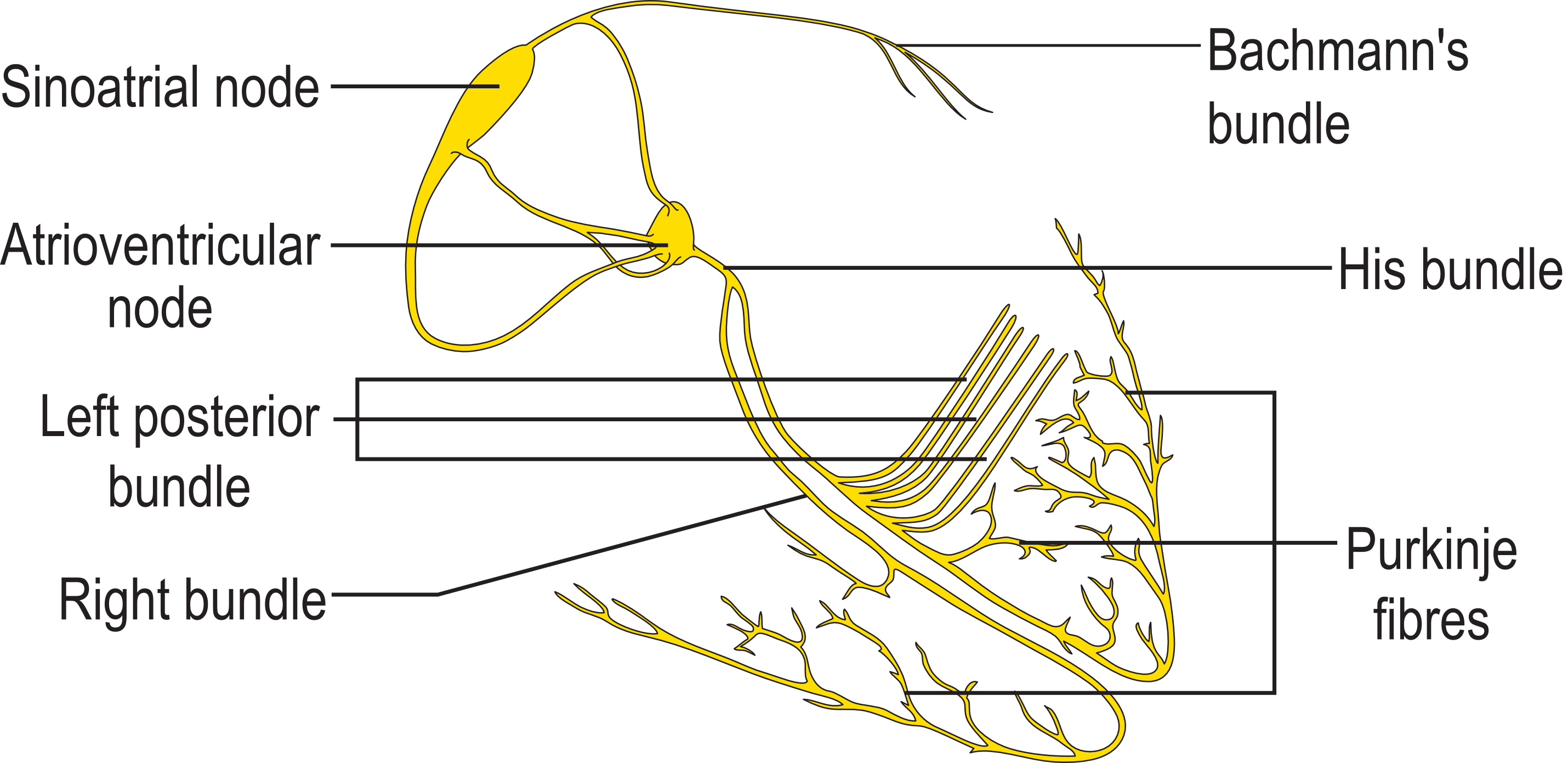
Interpreting an ECG trace
ECG or electrocardiography is a way of tracking the electrical activity of the cardiac muscles. ECG works by measuring changes in voltage due to polarization and depolarization of cardiac muscles through electrodes attached to the skin. A normal ECG trace have spikes marked with P, Q, R, S, and T. The P wave marks the depolarization of the SA node which results in systole of the atria. The big spike called the QRS complex marks the depolarization of the AV node and systole of the ventricles. Additionally, hidden in the spike is the repolarization of the SA node. Lastly, the T wave shows the repolarization of the ventricles. An ECG trace is useful since it is easy to detect abnormalities in a heart rhythm by using an ECG. For example, changes in heart rhythm can be detected easily by seeing that the frequency of spikes changes.
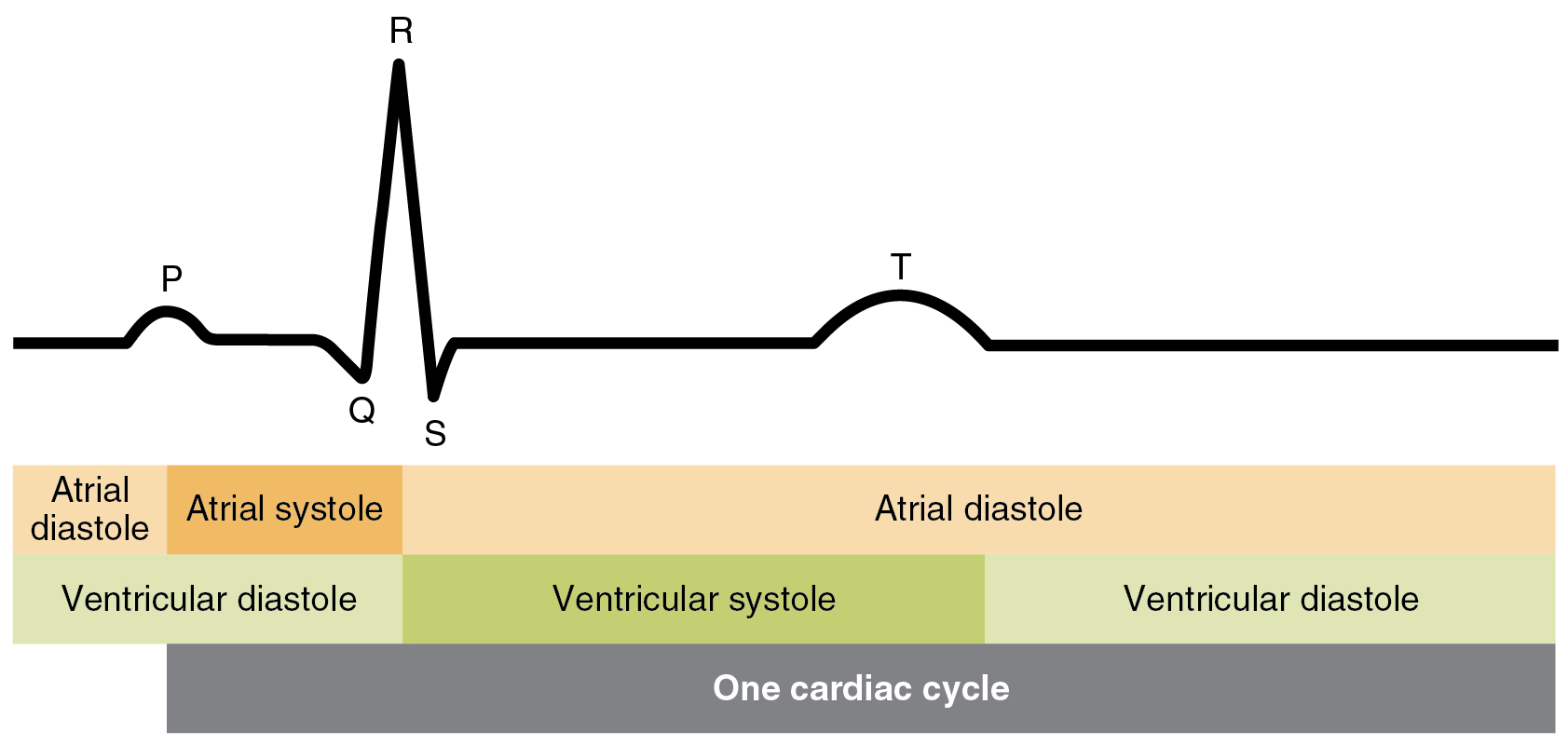
Common heart problems
Fibrillation and arrythmia arise when the SA node stops working normally, resulting in chaotic heartbeats too weak to pump blood throughout the body. Fibrillation is when the heart “shivers” as a result of weak and jumbled signals from the SA node, and arrythmia is when there is no consistent rhythmic pulse, thus messing up the timing between the atria and ventricles. Both conditions are often solved by providing an external source of electrical signals such as a pacemaker or by using a defibrillator. Defibrillators work by sending an electrical shock to the heart which in some cases is enough to restart the heart’s rhythm, making it beat normally again. In more serious cases when a defibrillator is not sufficient, a pacemaker may be necessary. A pacemaker provides an external SA node which mimics the SA node by sending electrical signals in regular intervals. However, a pacemaker has to be inserted by surgery and is powered by a battery which needs to be replaced from time to time (around 7 years). A pacemaker is thus only considered when absolutely necessary.
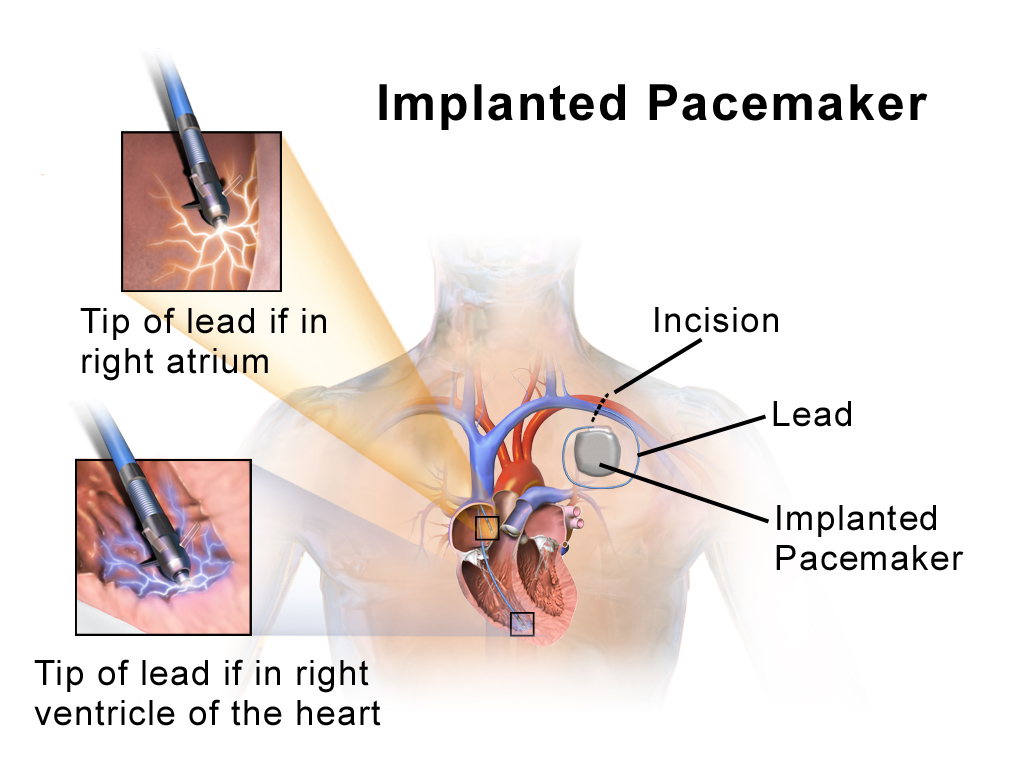
Thrombosis or blood clotting arises when platelets in the blood form a clot inside a blood vessel, thus stopping blood flow to a part of the body. If the blood clot is in a major vessel or a vessel supplying blood to an organ the consequences can be quite serious. A thrombosis in a vein supplying blood to the brain can cause a stroke, and a thrombosis in an artery supplying blood to the heart can cause a heart infarct.
Hypertension or high blood pressure is a condition where the blood pressure is higher than what is considered normal. Blood pressure is measured with two numbers such as 118/70. The “numerator” refers to the systolic pressure in the arteries, and the “denumerator” refers to the diastolic blood pressure. Both numbers have the unit mm Hg (millimeters of mercury) (approx. 133 pascals).
Visit this page for more information about blood pressure:
Understanding Blood Pressure Readings
The endocrine system
Most hormones are secreted trough endocrine glands directly to the blood stream. This allows the hormones to move throughout the body to reach their target cells. Contrary the exocrine glands, endocrine glands secrete their contents into the bloodstream instead of ducts. This means that all cells can come in contact with the contents, but the contents (usually hormones) only interfere with a specific type of cells called the target cells. An example of an endocrine hormone is thyroxin which is produced in the thyroid glands in the front of the neck under the Adams apple. Thyroxin plays an important role in regulating the rate of metabolism.
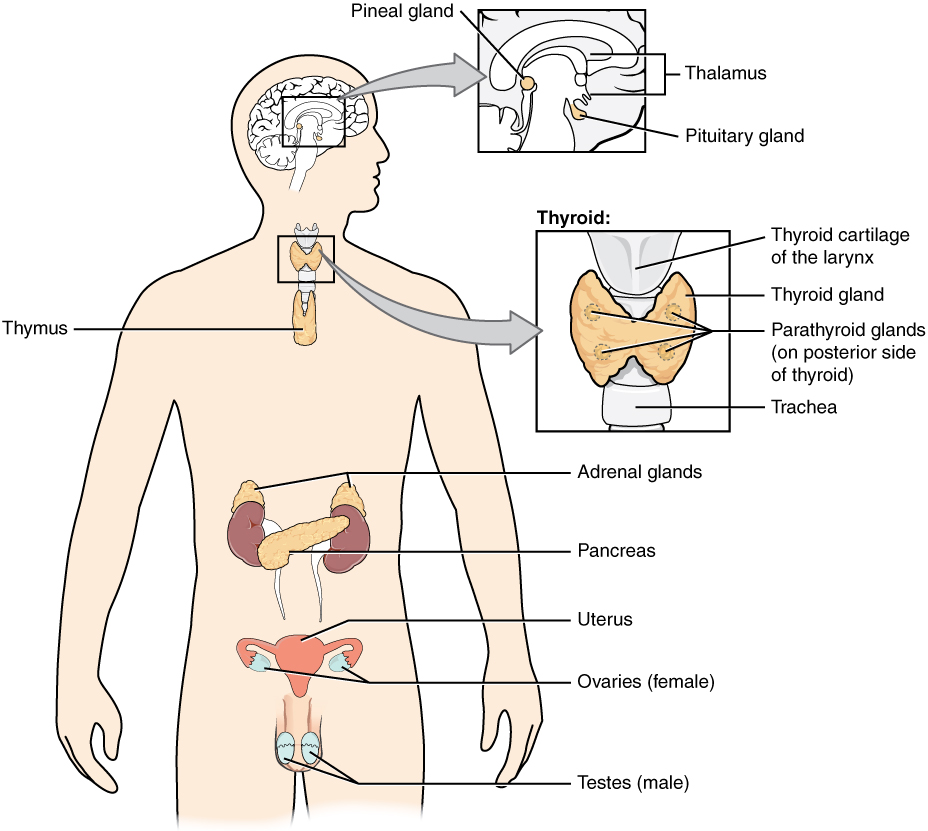
Steroid hormones
Steroid hormones all have similar chemical compositions which makes them characteristically lipid soluble. This means that they can easily pass through the phospholipid bilayer of cell membranes. Steroid hormones work by inhibiting or promoting production of proteins within target cells by entering the cell through the cell membrane and forming a receptor-steroid complex which enters the nucleus and binds to a specific gene. The complex can then control the production of the protein which the gene codes for. An example of a steroid hormone is testosterone which is the male sex hormone which regulates many of the developmental and reproductive properties of a male.
Peptide hormones
Peptide hormones like steroid hormones also inhibits or promotes the production of proteins within target cells by interacting with the DNA. However, peptide hormones are made of amino acids and are therefore not as lipid soluble as steroid hormones and therefore cannot enter the cell plasma as readily. Peptide hormones thus binds to surface receptors instead which carries chemical messengers inside the cell which gets released when a hormone binds to the surface. The chemical messenger may activate a chain of enzymes which at the end of the chain activates a transcription factor which enters the nucleus and interacts with the DNA.
Visit this page for information and diagrams of how hormones work:
The pituitary gland and the hypothalamus
The pituitary gland and the hypothalamus are responsible for a major part of the hormones released in the body. The pituitary is comprised of two parts, the posterior and anterior pituitary. The posterior pituitary has cells called neurosecretory cells which extend from the hypothalamus into the posterior pituitary. The cell bodies and dendrites are in the hypothalamus, but the axons extend into the pituitary. The hormones are produced in the cell bodies (inside the hypothalamus) and then released from the axons (in the posterior pituitary). It is thus the case that the posterior pituitary gland only releases hormones which they in reality are produced in the hypothalamus.
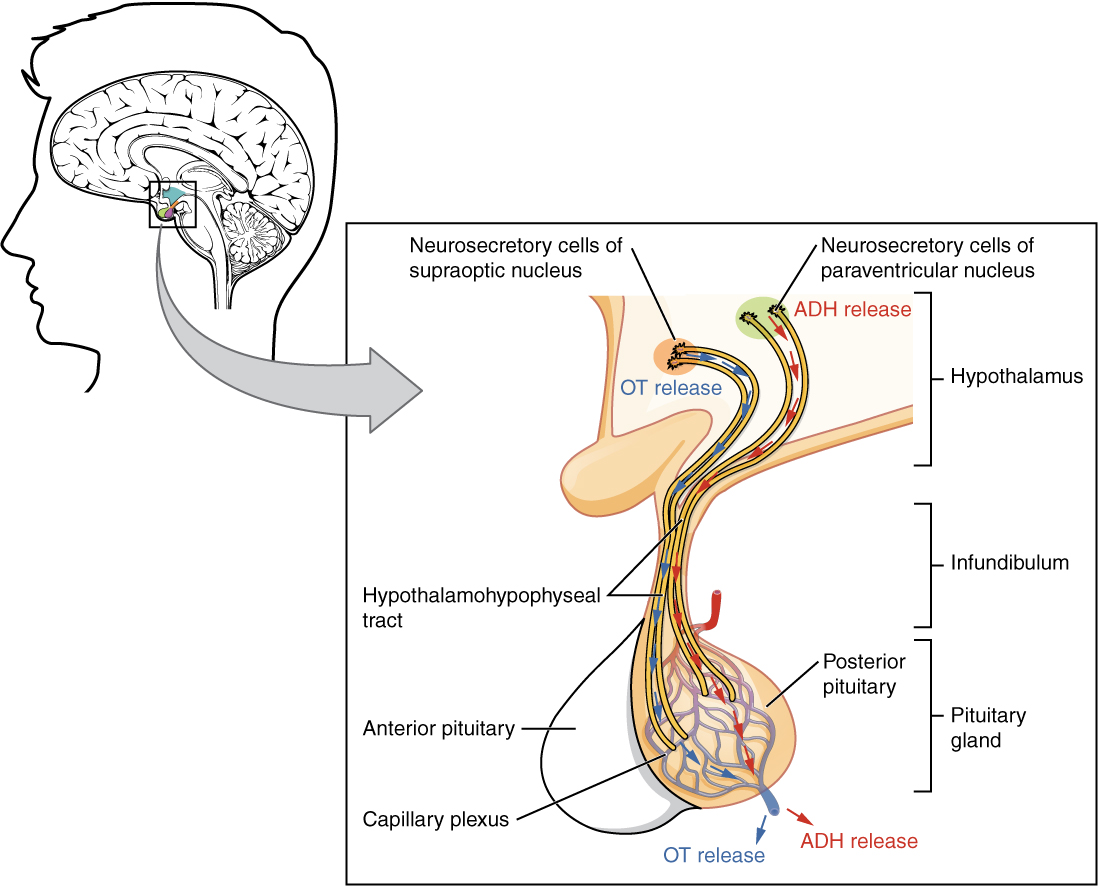
The anterior pituitary is similar to the posterior pituitary in that they both receive their hormones from the hypothalamus. However, in the case of the anterior pituitary, there are no neurosecretory cells. Instead, the hormones produced by the hypothalamus are released into the blood stream through a capillary bed which is connected to a capillary bed in the anterior pituitary by a portal vein. The hormones produced by the hypothalamus which are sent to the anterior pituitary are releasing hormones, for example gonadotropin releasing hormone. Releasing hormones regulates the secretion of other hormones by the anterior pituitary, for example luteinizing hormone (LH) and follicle stimulating hormone (FSH).
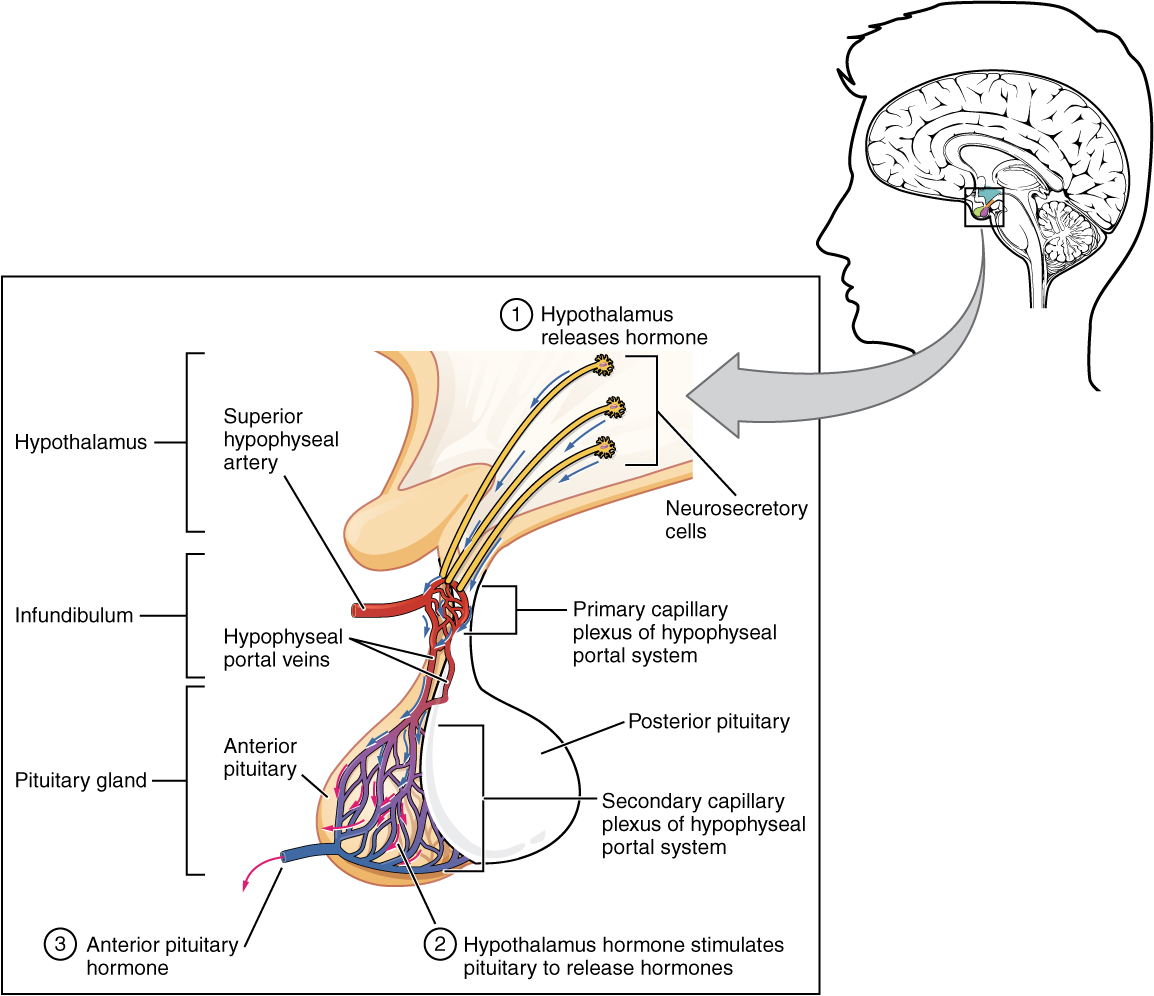
The posterior pituitary can thus be seen as a storage unit, receiving hormones produced by the hypothalamus to get released from the posterior pituitary. The anterior pituitary is more of a factory, receiving instruction from the hypothalamus and then assembling the hormones in the anterior pituitary which it then secretes into the blood stream.
Hemoglobin and oxygen affinity
Red blood cells are made of millions of hemoglobin molecules which are made of 4 haem groups each. The job of red blood cells is to carry oxygen around the body, but the component within red blood cells which carries the oxygen is the haem groups within hemoglobin. Haem groups have a central iron atom which is capable of carrying 1 oxygen molecule each, and since hemoglobin has 4 haem molecules it can carry 4 oxygen molecules. However, the affinity for oxygen changes with its saturation. It is proposed that hemoglobin can change its shape depending on how many oxygen molecules are bonded to the haem groups which in effect changes its affinity. The affinity for oxygen is lowest when hemoglobin does not carry any oxygen atoms. The affinity then increases with each bonded oxygen molecule, reaching a maximum at 3 oxygen molecules until it plummets when it is fully saturated at 4 oxygen molecules.
Other factors which effect the saturation of hemoglobin molecules is the oxygen pressure and concentration of other gases such as CO2. When oxygen pressure increases, saturation increases as expected because of the collision theory. When pressure increases, successful collisions increase which in this case means that more oxygen atoms bind to haem groups. When CO2 concentration increases, oxygen saturation decreases since hemoglobin can also bind to CO2, which creates a competitive scenario where CO2 may take the place of oxygen, thus decreasing oxygen saturation.
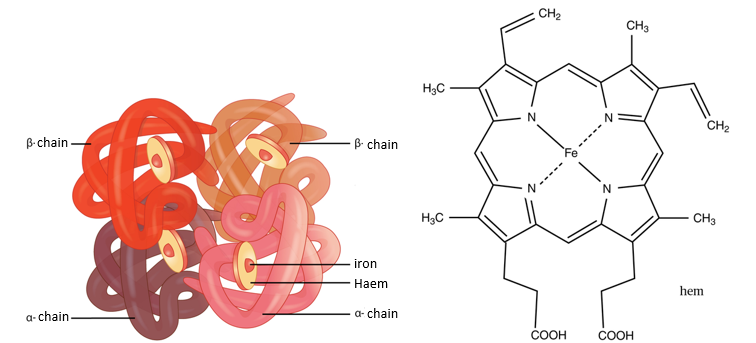
Oxygen dissociation curves and Bohr shift
Oxygen dissociation curves can be used to visualize the affinity of hemoglobin for oxygen in addition to predicting how much oxygen gets released as pressure varies. The x-axis shows the oxygen partial pressure, while the y-axis shows oxygen saturation. At high pressures hemoglobin is fully saturated, while it increases in a “s” shape at lower pressures. The non-linear increase shows that the affinity for oxygen changes with saturation. From the graph it is possible to predict the oxygen released when pressure changes by looking at the change at the y-axis. When inhaling, oxygen pressure increases which increases the saturation of hemoglobin molecules. When these saturated hemoglobin molecules reach areas with low oxygen pressure such as in respiring cells, the saturation decreases. The difference in saturation can be used to calculate the amount of oxygen released.
The same phenomenon can be explained with carbon dioxide concentrations. The CO2 concentration is low in the lungs which means that hemoglobin has a stronger affinity for oxygen. However, when reaching respiring cells, the CO2 level increases which causes some hemoglobin to bind to CO2. This decreases hemoglobin’s affinity for oxygen and thus reduces saturation. The difference between the oxygen dissociation curve in the lungs and in respiring tissues is called the Bohr shift.
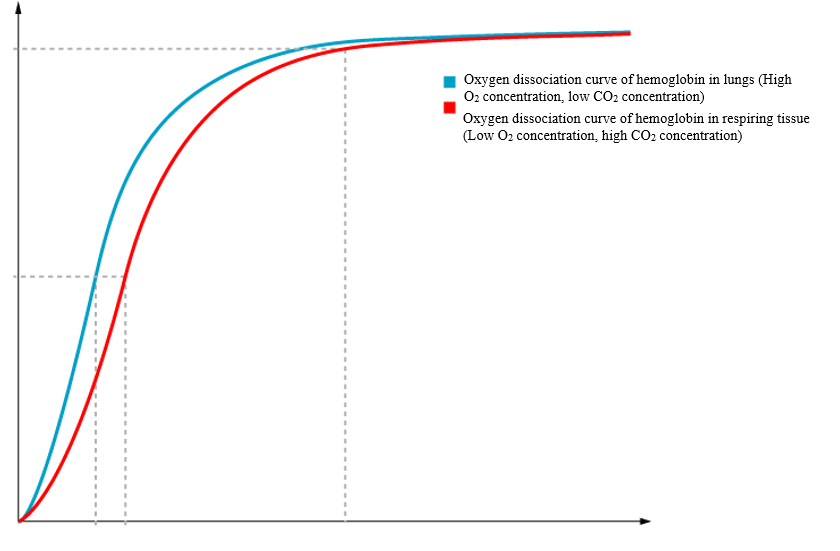
Dissociation curves of myoglobin and fetal hemoglobin
Myoglobin is similar to hemoglobin in that it is an oxygen-binding protein. However, the proteins are in muscle cells and their function is to provide a last resort of oxygen during anaerobic respiration. Myoglobin thus needs to be able to hold on to its oxygen until the pressure gets so low that it is basically anaerobic. This can be confirmed by looking at its dissociation curve.
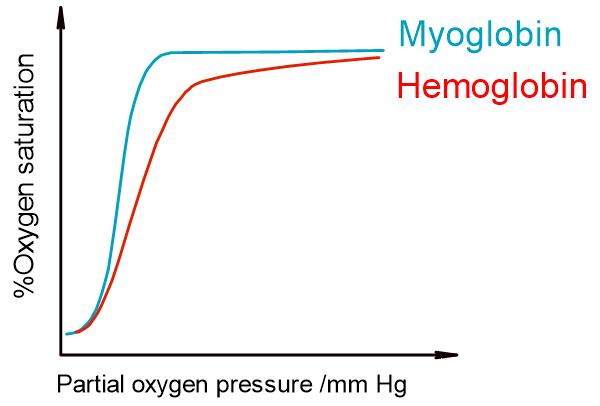
Fetal hemoglobin is very similar to adult hemoglobin except that it has a stronger affinity for oxygen than adult hemoglobin. This is to ensure that oxygen is dissociated across the placenta so that the fetus gets a supply of oxygen. One analogy that can be used is that fetal hemoglobin is a stronger oxygen magnet than adult hemoglobin. When adult hemoglobin comes along with an oxygen molecule, the stronger magnet will take the oxygen molecules away from the weaker magnet.
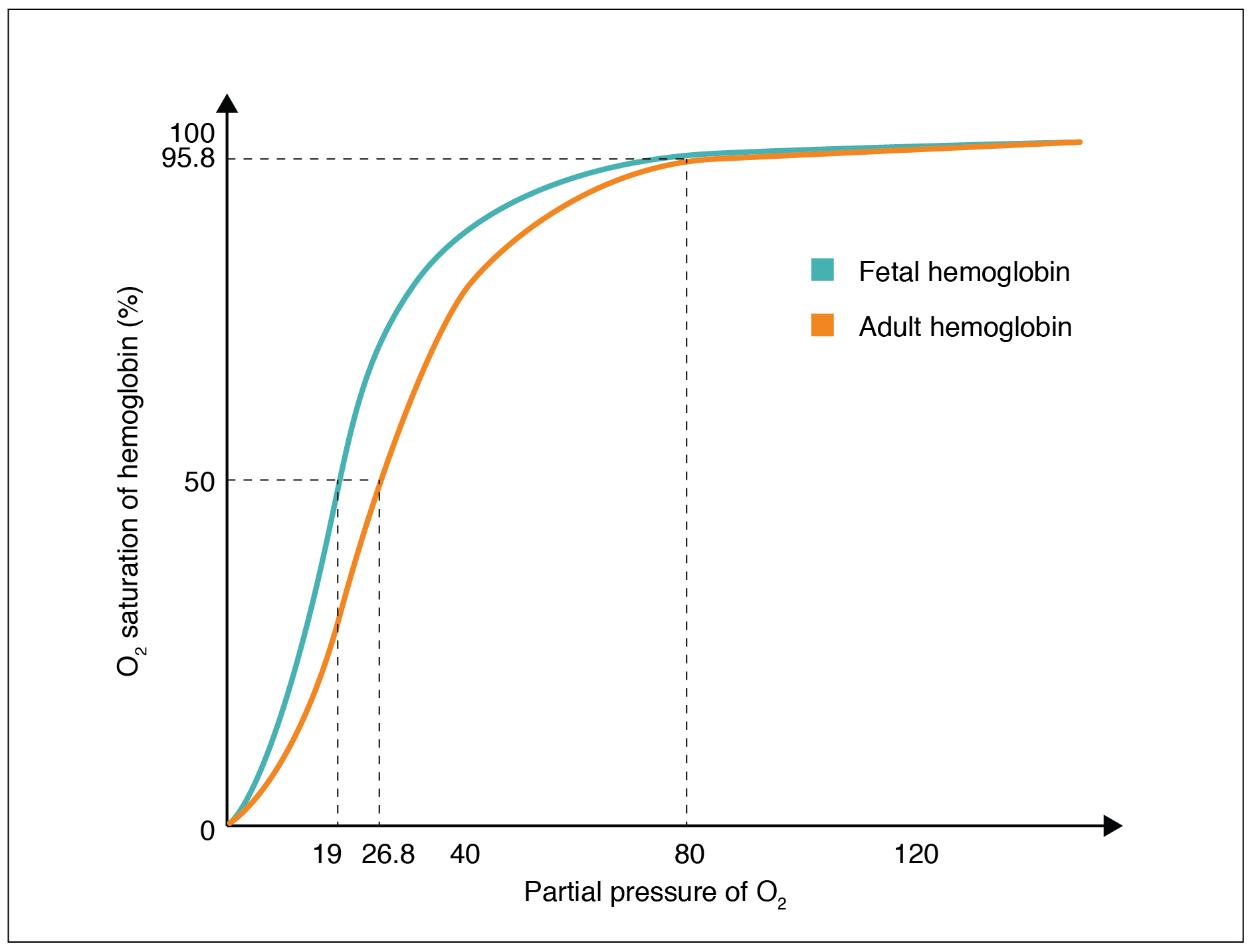
Carbon dioxide transport
Carbon dioxide can be cleared in three ways. A very small percentage dissolves in the blood plasma, a larger percentage is bonded to hemoglobin and leaves through expiration, but the largest percentage (approximately 70%) is turned into carbonic acid (H2CO3). Enzymes in the cytoplasm of red blood cells called carbonic anhydrase catalyzes a reaction between water and carbon dioxide to create carbonic acid which dissociates into HCO3- and H+. For those taking chemistry it may be familiar that an increase in H+ concentration lowers the PH. Since PH levels need to be held consistent at around 7.35-7.45 the body buffers the PH by “absorbing” H+ ions. H+ can be buffered either by bonding to hemoglobin before it leaves the red blood cell, or bonding to various proteins in the blood plasma after leaving the red blood cell. Both of the cases remove H+ from the solutions and buffers the PH. HCO3- leaves the cells by a chloride shift. The cell exchanges one HCO3- for a Cl-, thus preserving charge on both sides. HCO3- can then be discarded through urine.
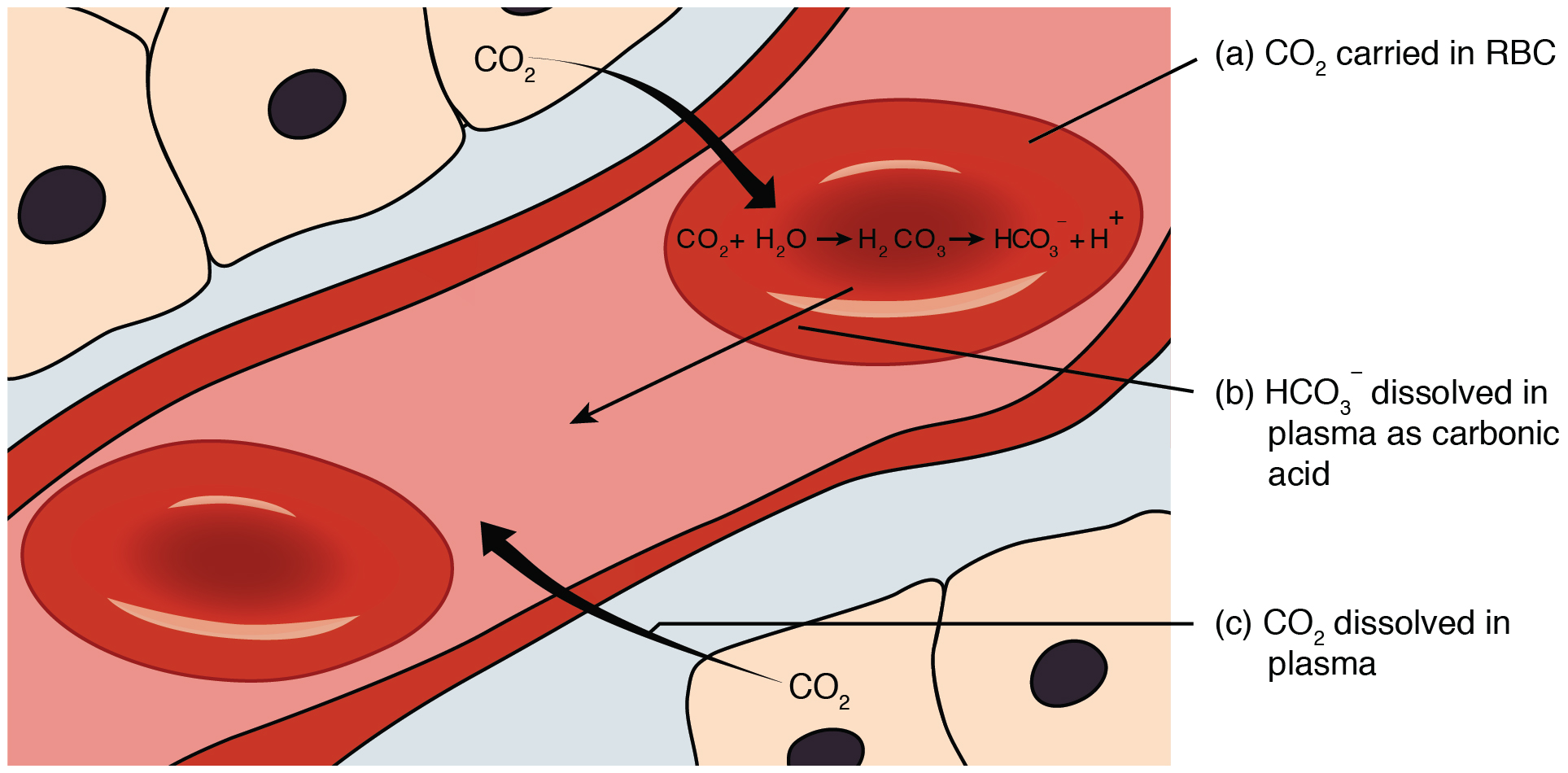
Control of respiration rate
When exercising the PH levels decreases as the skeletal muscles expend glucose to produce ATP and CO2. The CO2 then turns into carbonic acid which dissociated into an H+ ion thus decreasing PH. When receptor cells called chemosonsors detects this decrease it sends action potentials to the medulla oblangata which then sends action potentials to increase the respiration rate. After activity has seized and the chemosensors detects an increase in PH, it sends signals to reduce respiration again.
Helpful videos:
The liver: https://www.youtube.com/watch?v=wbh3SjzydnQ
Excellent video on the cardiac cycle and ECG's: https://www.youtube.com/watch?v=EMmjwgwHkO0
The pituitary gland and the hypothalamus: https://www.youtube.com/watch?v=xCXtcoUxJZc
Hemoglobin-Oxygen dissociation curves: https://www.youtube.com/watch?v=HYbvwMSzqdY
I found this topic to be one of the easier since it really does not add too much to previous knowledge. Either way it was an interesting read, and I definitely did learn something. Finishing this option marks the end of the Biology curriculum and this website. All in all, I really liked the course and I think it covers mostly all of the general biology taught at high school level. I think you should be very happy with yourself if you managed to finish the course. Hopefully you have gained an interest in the subject to continue exploring biology on your own and maybe even pursuing a career in the field😊