Physiology is the study of the functions and activities of life, and the physical and chemical processes involved. Topic 6 investigates a variety of systems in the human body, namely the digestive, circulatory, respiratory, immune, nervous, and hormone system. It is a long and difficult topic, but it is in my opinion also one of the most interesting topics. The number of names and terms in this topic may be daunting. However, it gets easier with the right approaches. Visualization and labeling of the different components in the different systems is really the key to this chapter. Make a map of each system and try to visualize how the different components in the system interacts to carry out its function. For anyone taking the BMAT like me, this is the chapter you should pay extra attention to.
Points for revision:
- The digestive system anatomy
- Heart anatomy and blood flow
- The immune system and primary defense
- Primary and secondary response
- The respiratory system anatomy, alveoli, and gas exchange
- The hormone system and regulation of blood glucose levels
The digestive system anatomy
Digestion is the process of converting big food molecules into their smallest units so they can be transported through the blood system. The digestive system is essentially a long tube called the alimentary canal connected to two accessory organs, the pancreas and the liver. The alimentary canal is a long muscular tube that starts in the mouth and ends with the anus. It has two layers of muscles that moves food down the digestive tract in an action called peristalsis. The two layers of muscles are divided into the inner circular muscles and the outer longitudinal muscles. The cavity within the alimentary canal is called the lumen. Furthermore, the alimentary canal in the small intestine has an inner membrane lined with villi.
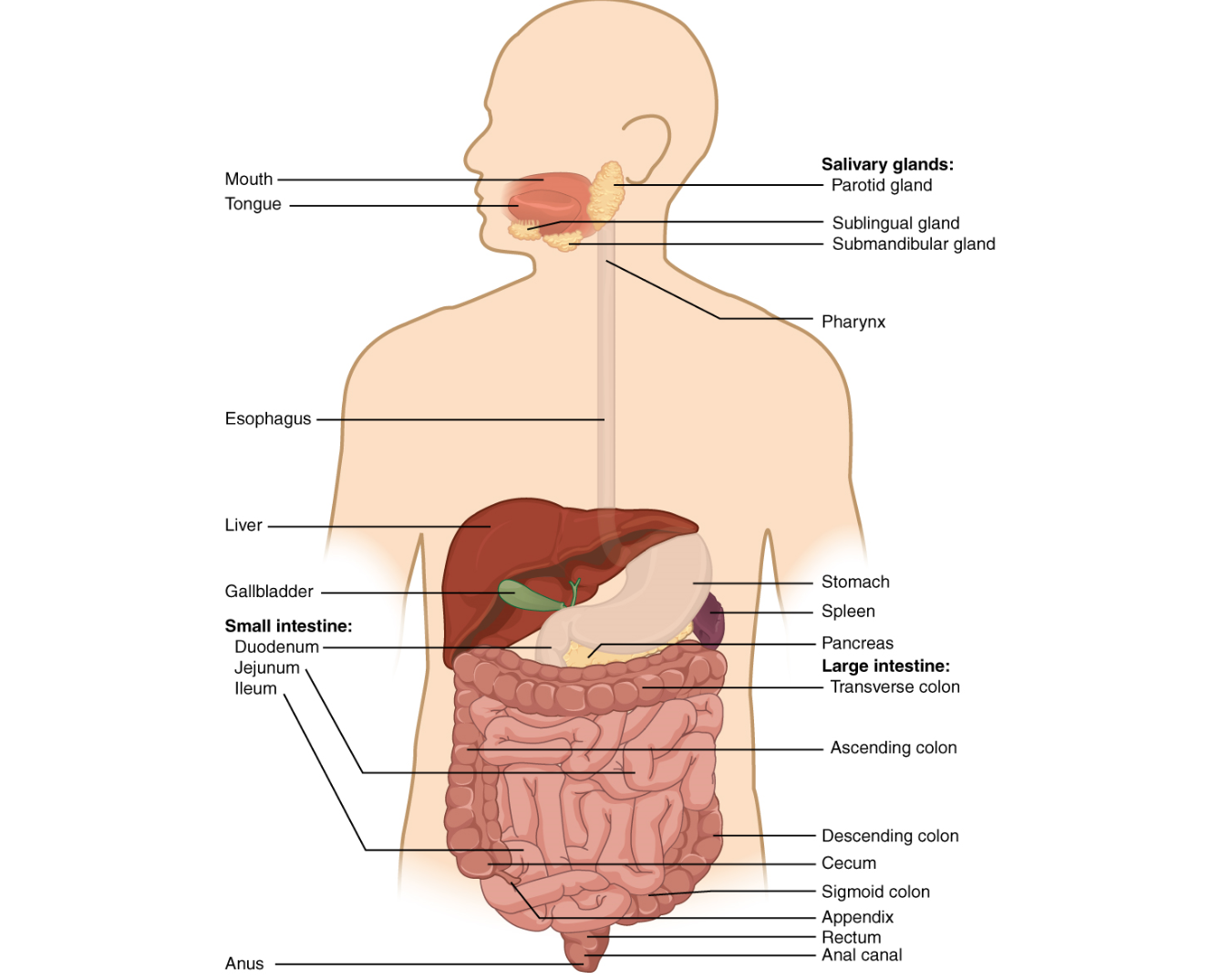
Intestinal villi
Villi are small tissue projections that greatly increases the inner surface area of the small intestine and are important in the absorption of nutrients. Each villus is connected to the blood system and the lymph system trough its inner capillary bed and lacteal. When food in the lumen comes in contact with a villus, its single cell layered epithelial allows for diffusion into the capillary bed and lacteal, thus transporting the nutrients to the rest of the body. The molecules that cannot be diffused are transported into the villi by facilitated diffusion or active transport. Examples can be polar molecules like glucose, or molecules moving against its concentration gradient like glucose under certain circumstances.
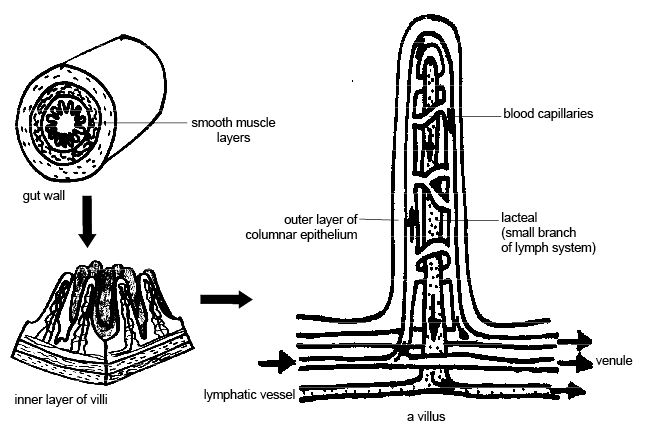
Enzymes in digestion
The actual process of digestion is catalyzed by enzymes that breaks down big nutrient molecules into their smallest units so they can diffuse into the villi. Carbohydrates are hydrolyzed into monosaccharides, proteins into amino acids, and lipids into glycerol and fatty acids. We will start by looking at carbohydrates.
Carbohydrates in food are usually found as starch or sugars. Starch molecules are big molecules made of long chains of glucose molecules bonded into a branched structure. The first enzyme involved in digesting carbohydrates is called amylase. Amylase is secreted from the salivary glands into the mouth, and pancreatic amylase is secreted into the duodenum by the pancreas. Amylase breaks big carbohydrate molecules like starch into the smaller disaccharide maltose. Maltose is then further broken down into glucose by another enzyme called maltase in the intestine.
Proteins are broken into polypeptides and amino acids by two enzymes called pepsin and trypsin. Pepsin is found in the stomach and starts the digestion of proteins by hydrolyzing them into polypeptides. However, since pepsin has a peak reactivity in acidic PH levels, its reactivity is greatly reduced in the higher PH levels in the duodenum. Another enzyme called trypsin then takes over in the small intestine, breaking down the polypeptide chains into amino acids.
Lipids are broken down into glycerol and fatty acids by an enzyme called lipase. However, the hydrophobic properties of lipids prevent it from mixing with the watery chyme in the stomach. This is a problem because the enzymes only get a small surface area to work with, which leads to limited reactivity. The lipids therefore must go through a process called emulsification. In emulsification enzymes from bile produced by the gall bladder breaks down the lipids into smaller parts, thus increasing its surface area and the rate of reactivity.
The blood system anatomy
The blood system is the transportation system of living organisms and it is comprised of blood vessels and the heart. Blood vessels are divided into arteries, veins, and capillaries. Arteries transport blood away from the heart, and veins transport blood into the heart. Capillaries are thin blood vessels involved in the exchange of material between tissues and the blood. Arteries and veins are further divided into smaller vessels called arterioles and venules. Blood pumped out of the heart usually follows the route: artery, arteriole, capillary bed, venule, and vein. Because of the pressure from each heartbeat, arteries need to have thick walls to withstand the pressure delivered from the heart. Veins on the other side, do not need to have thick walls because of the low internal pressure. However, the low pressure makes it possible for blood to flow back, so veins have internal valves to prevent backflow. All exchange of material happens in the capillaries, as the walls of the arteries and veins are too thick for diffusion to occur.

Heart anatomy and blood flow
The heart is a pump with four chambers made of cardiac muscles that continuously makes sure that blood is flowing throughout the body. It can be divided into the left and right side, with the left side being called the systemic circulation, and the right side being called the pulmonary circulation.
Blood enters in the right side through the superior and inferior vena cava and flows into the right atrium. Both the left and right sides of the heart have atriums and ventricles. The atrium functions as a collection chamber for blood and is separated from the ventricle by an atrioventricular value. The ventricle is the powerhouse of the heart. Its thick muscled walls can create a lot of pressure, thus pumping blood out of the heart. Deoxygenated blood flows in from the right side and is pumped out from the pulmonary arteries into the lungs. Oxygenated blood returns through the pulmonary veins of the left side and is finally pumped to the organs through the aorta.
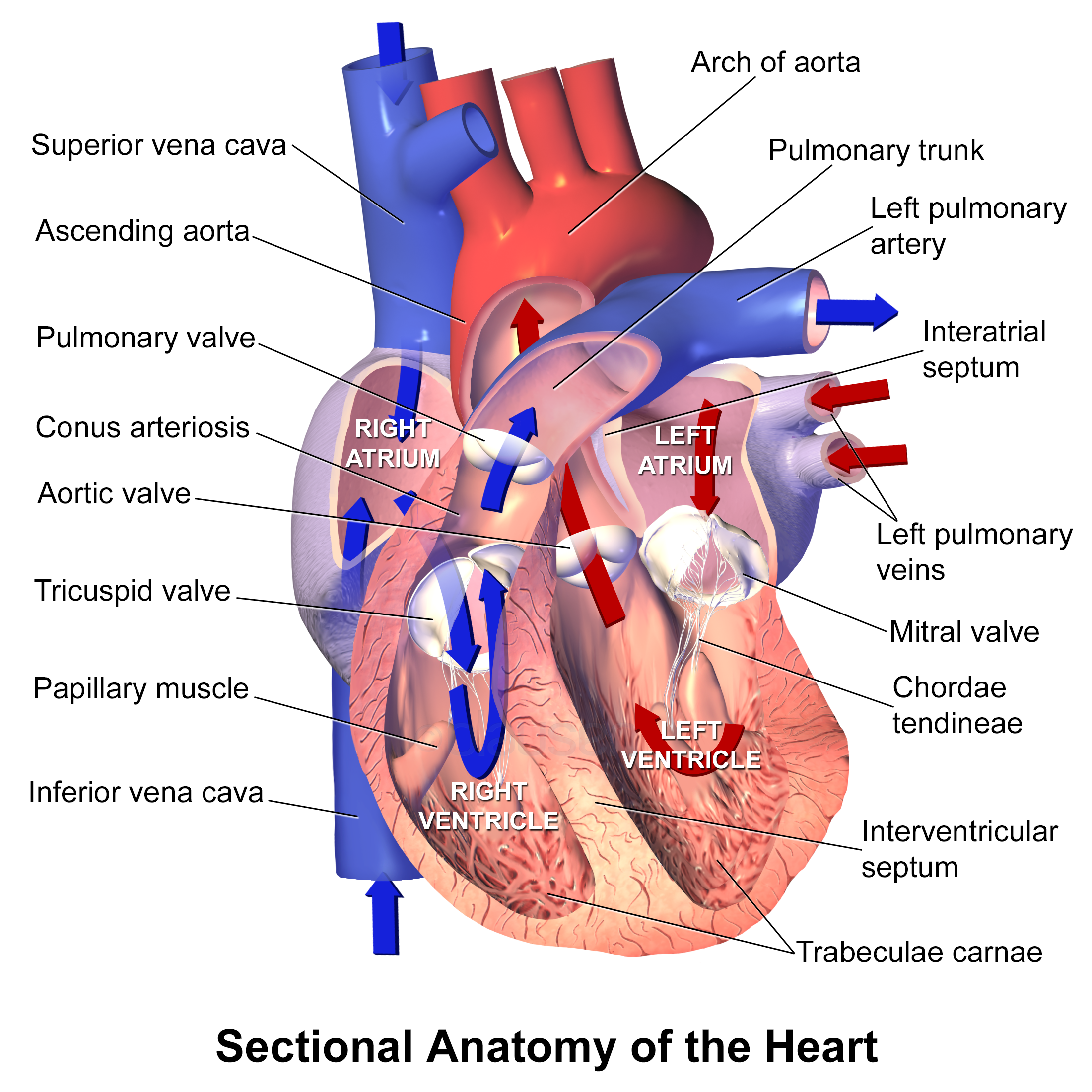
Note: The IB refers to the valves as the left and right atrioventricular valves and left and right semilunar valves. Other literature can have other more specific names for these valves.
Control of heart rate
The heartbeat is controlled by the sinoatrial node and the medulla. The right atrium is comprised of a specialized tissue called the sinoatrial node (SA node). The sinoatrial node functions as a pacemaker, sending out a signal in even intervals, telling both atria to contract. After the initial signal, the SA node then sends a delayed signal to the atrioventricular node (AV node), telling both ventricles to contract. This creates a rhythmic heartbeat. However, during exercise, an increased rate of respiration increases carbon dioxide production and the demand for oxygen. The medulla in the brainstem senses an increase of carbon dioxide in the blood, and sends a signal down the cranial nerve, telling the SA node to send signals at a faster rate thus increasing the heart rate. When carbon dioxide levels fall again, the heart rate slows down to normal.
Pumping blood
Valves in the heart prevent backflow by only allowing blood to flow in one direction. When a chamber in the heart is contracting, it is said by be systole, and when it is not contracting, it is said to be diastole. The exchange between a diastole and systole chamber is important in deciding whether the value is open or not. When both the atrium and the ventricle is in diastole, the atrium has a higher pressure that the ventricle, thus opening the atrioventricular value and letting blood flow passively. When the atrium is systole and the ventricle is diastole, the pressure difference increases even more, thus pumping all the blood from the atrium to the ventricle. Throughout all this, the pressure in the aorta is maintained quite high, thus shutting the semilunar valve, and preventing flow from the aorta. However, due to the muscular tissue surrounding the ventricle, it quickly builds up pressure when it is systole. In early ventricular systole, the pressure in the ventricle exceeds the pressure in the diastole atrium, thus shutting the atrioventricular value, and preventing further flow from the atrium. The semilunar valve is still closed, and it is first under late diastole that the pressure in the ventricle exceeds the pressure in the aorta, thus opening the semilunar value and pumping blood out of the aorta. When the blood is pumped out, the pressure falls and both chambers go back to be diastole, starting the cycle over again. It is the closing and opening of these valves that makes the heartbeat sound.
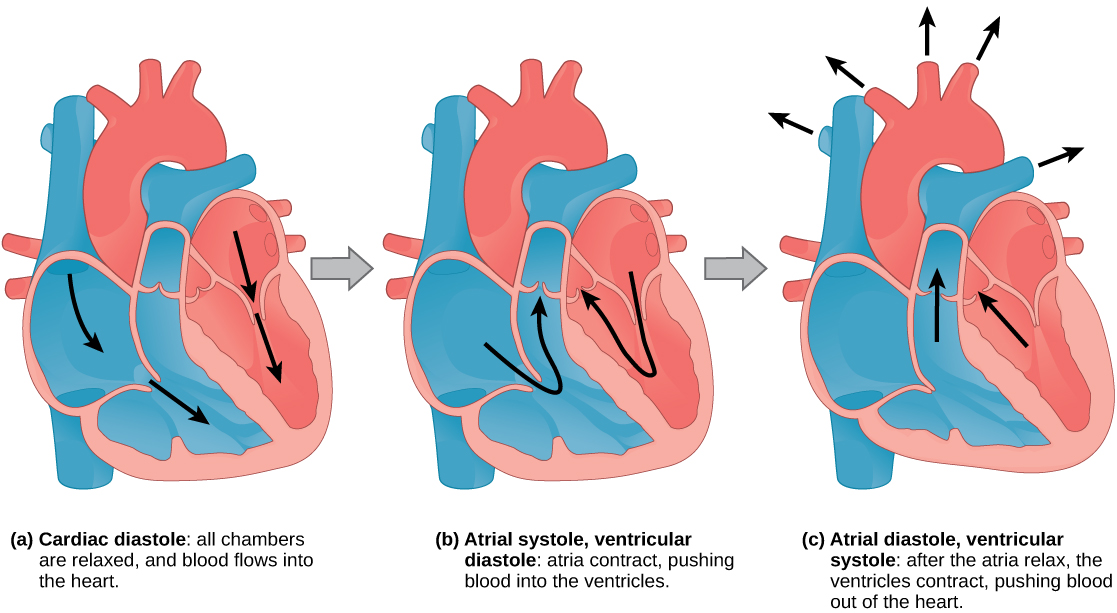
The immune system and primary defense
The goal of the immune system is to keep pathogens out of our body, and when it fails to do so, it initiates a series of responses targeted at killing the invader. The primary defense of the body is the skin. Pathogens replicate and pass on their genetic material by invading living cells and using them as pathogen factories. However, since the outer layer of the skin (called the epidermis) is composed of dead cells, pathogens cannot infect it. The epidermis thus functions as a shield, protecting all the underlying tissue. Unfortunately, not all the body is protected by skin. Openings such as the mouth and the nose are vulnerable to intruders since they are not protected by anything. The body has therefore developed mucous membranes to protect these openings. Mucous membranes secrete mucus, which is a sticky substance that can trap invading pathogens, preventing them from reaching their target cells. Hairlike structures called cilia moves the mucus and the trapped pathogens out of the openings, thus preventing disease. A pathogen is any living organism or virus that can cause disease.

Blood clotting
When pathogens move past the primary defenses, for example through a cut, a series of processes are put in motion. Firstly, the cut must be clotted to prevent blood loss and more pathogens from entering. When a blood vessel is damaged, it releases chemical substances in the blood stream. These signals trigger platelets and plasma proteins to adhere to the area. The two plasma proteins involved in blood clotting are called prothrombin and fibrinogen. When the plasma proteins arrive at the target area, the chemicals released converts prothrombin into thrombin, which then converts fibrinogen into fibrin. Fibrin has a mesh like structure that traps the platelets, thus sealing the cut and preventing further infection. Platelets are broken up dead cells produced in the bone marrow.
Immune response
The immune system now has to deal with the pathogens already in the body by initiating the immune response. The two primary cells involved in the immune response are the white blood cells (leucocytes) and the plasma cells. White blood cells in the form of macrophages fights invading pathogens by devouring them through phagocytosis, and then disabling them by chemical digestion. A macrophage can use the surface proteins of cells and viruses to detect if it is self or not-self. If it is not-self, the phagocyte will devour it by changing its shape and engulfing it. Because of the shape changing properties of macrophages, they can squeeze themselves through blood vessels and thus initiate an attack outside of the blood vessels, stopping the pathogens early on. In addition to macrophages, the immune system has a second line of defense comprised of plasma cells. Plasma cells can produce Y shaped antibodies that has two binding sites on its “claws”. These binding sites are specialized to match the shape of only one type of antigen. An antigen is a cell or virus determined as not-self. When the antibody binds with a matching antigen, the antibody disables the pathogen or marks it for destruction by other cells. Each type of plasma cell can only produce one type of antibodies, and each type of antibody only works on one type of antigen.
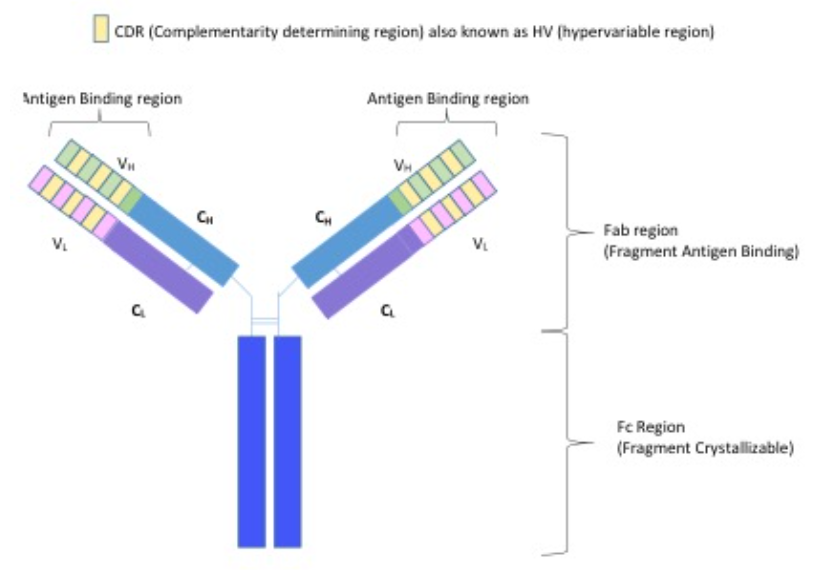
Primary and secondary response
The first time a pathogen is detected is called a primary response. In a primary response the body does not yet have any plasma cells that can produce the correct antibodies. The pathogens are therefore given a lot of time to replicate before the body can effectively defend against them. This is why some diseases can have severe symptoms and last for a few weeks the first time it is contracted. Fortunately, the body usually manages to find plasma cells that can produce the right antibodies, and when this happens, the plasma cell rapidly replicates thus creating an army of antibodies. When the pathogen is defeated, some of the plasma cells remain in the blood stream as memory cells, preparing for the next invasion. The next time the same pathogen attacks, a secondary response is initiated. The memory cells from the first infection can quickly produce antibodies, stopping the invasion before it can do any harm. Secondary responses are the reason why we can stay healthy most of the time, and it is the mechanism behind vaccines.
The respiratory system anatomy, alveoli, and gas exchange
The respiratory system is comprised of the lungs, the airways, blood vessels, and the muscles involved in breathing. The respiratory system oxygenates the blood flowing from the pulmonary artery, so that it can be transported to the rest of the body for aerobic respiration. The lungs are made of small air sacs covered with capillaries called alveoli. When these sacs fill up with air, oxygen moves into the blood and carbon dioxide into the alveoli by simple diffusion. This is made possible by the thin layer of pneumocyte type I. Alveoli are made of two types of pneumocytes, type I and II. Pneumocyte type I has a big surface area, and as previously mentioned, the single cell layer makes it easy for material to diffuse in and out. The second type of pneumocyte, pneumocyte type II, secretes a solution acting as surfactant that prevents the inner calls of the alveoli from sticking together. This makes breathing easier. Pneumocyte type I is incapable of mitosis, so when the cells die they do not have the ability to replenish themselves.
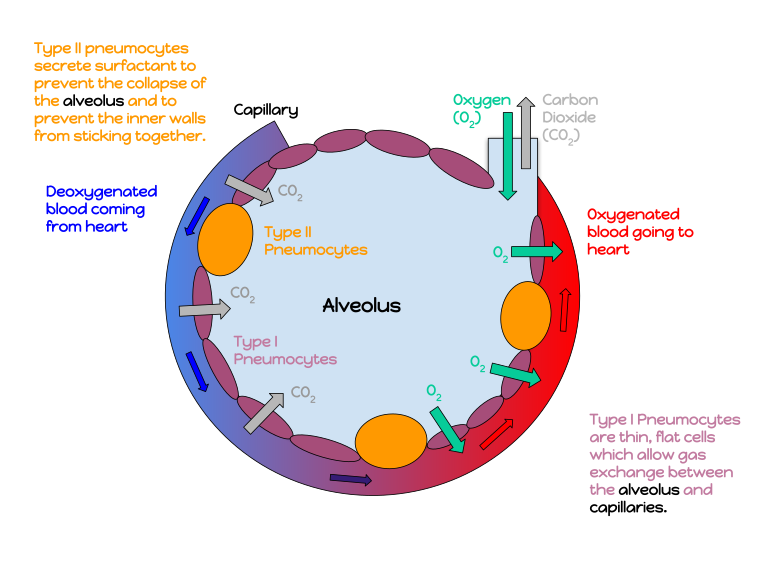
Inhalation and exhalation
The lungs are essentially a collection of small bags without any muscular tissue, so it is incapable of contracting on its own. It is therefore dependent of the surrounding muscle tissue to contract and expand. During inhalation, the lungs are expanded by the diaphragm moving down, and the external intercostal muscles contracting. The intercostal muscles lie on both sides of the ribs. When the diaphragm relaxes and the exterior intercostal muscles contract, the resulting partial vacuum in the lungs forces air to flow in. When the opposite action happens, that is when the diaphragm and the inner intercostal muscles contract, air is pushed out of the lungs, thereby exhaling. Air flows into the lungs through the trachea, which splits into two bronchi. The left and right bronchi further splits into bronchioles connected to the alveoli.
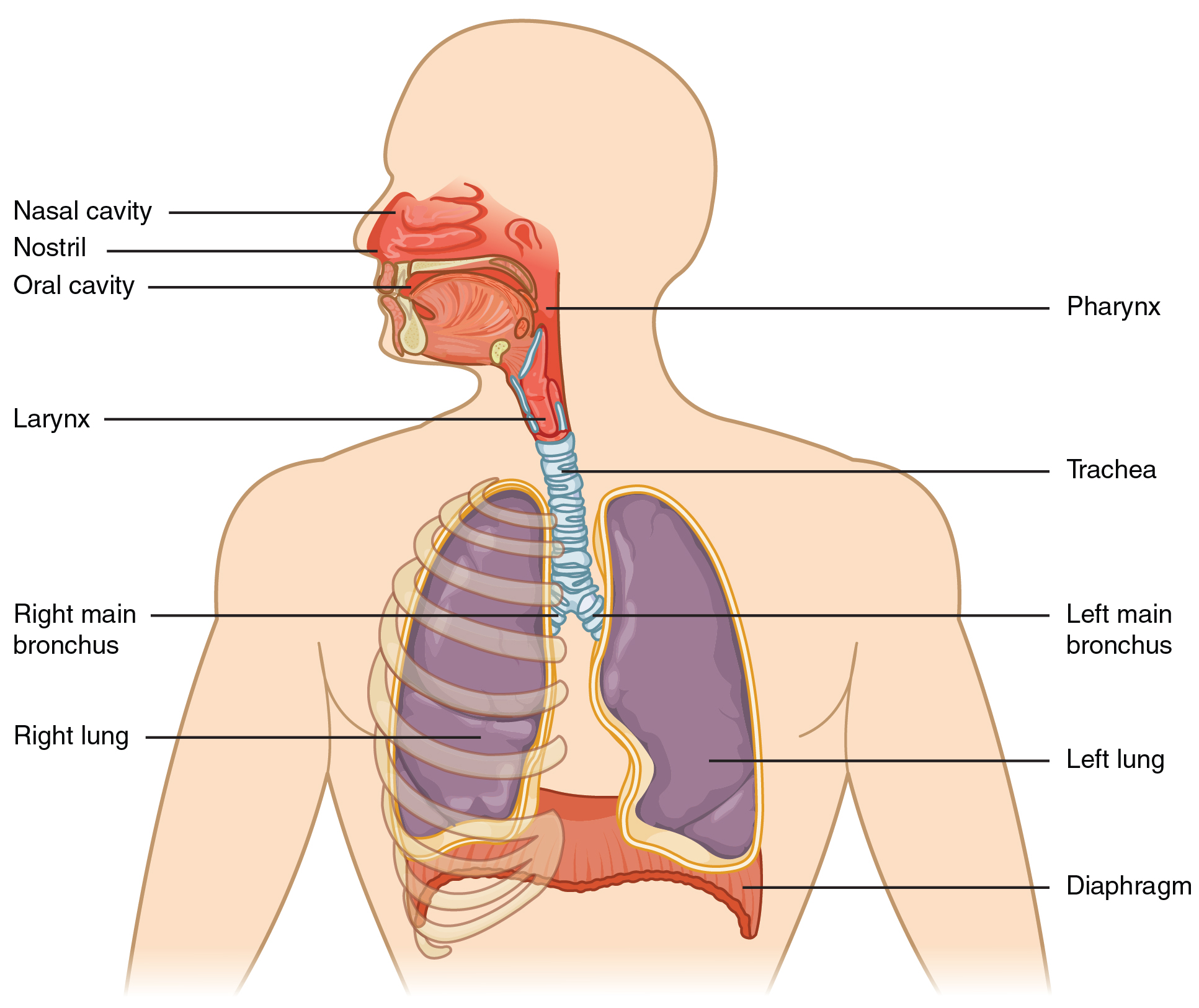
The nervous system
The nervous system is basically the communication system in the body. It allows the brain to communicate with the rest of the body by sending signals through neurons. The nervous system is comprised of the central nervous system, and the peripheral nervous system. The central nervous system is made up of the brain and spinal cord, and the peripheral system is made of all the nerves extending from the spinal cord. The nerves in the central nervous system are split into spinal nerves and cranial nerves. Spinal nerves extend directly from the spine, and cranial nerves extends directly from the brain stem. The nerves in the peripheral nervous system are split into motor and sensory nerves. Motor nerves sends signals from the spine to the target tissue, while sensory nerves send the signals from sensory tissue to the spine.
Neurons
Neurons are cells specialized to send electrical impulses. They are the longest cells in the body and can extend from the spine all the way to the foot. Neurons are made of a cell body with a nucleus and are connected to dendrites and an axon. Dendrites are like the input of the neuron. They are relatively short, but numerous, and they form branching structures so they can receive multiple signals at once. The axon is the output of the neuron, and it is the axon that can extend very far from the cell body. The axon can be covered by a sheet made of Schwan cells called myelin. Myelin is divided into segments called nodes of Ranvier that makes for more efficient neurons. The axon ends in a branching structure called the synaptic terminal, and each end of the branching structures has a terminal button. The terminal buttons can communicate with the next neuron through a synaptic gap between the terminal button and the dendrites of the next neuron.
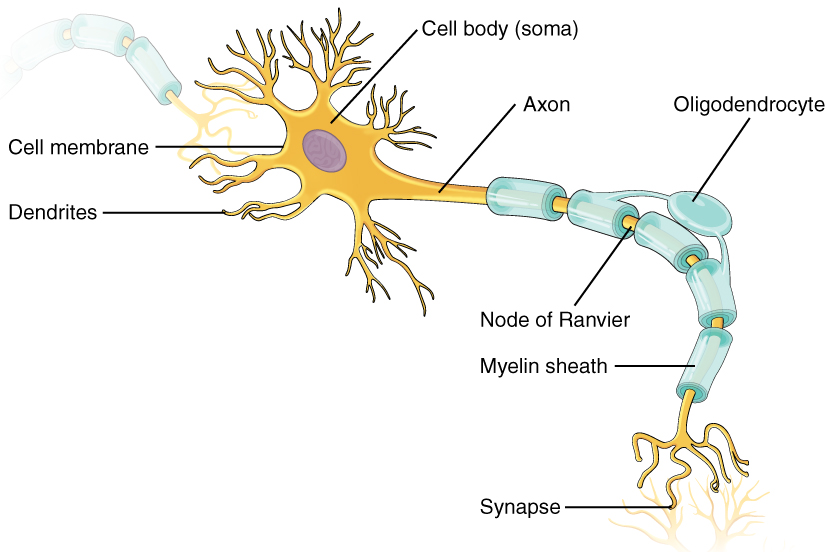
Resting potential
A nerve impulse is created by stimuli detected by sensory tissues that changes the electrochemical gradient in the neuron. When a neuron is not firing a signal, it is said to have a resting potential. During the resting potential, sodium potassium pumps transport material through active transport, creating a net negative charge inside the neuron. Sodium potassium pumps can change its shape by an expenditure of ATP. When an ATP molecule is bonded to the pump, one phosphate group is hydrolyzed, and the resulting energy changes the shape of the pump. The sodium potassium pump transports three sodium ions out of the cell, and two potassium ions into the cell by using the ATP to change its shape. A more positive charge outside of the cell is said to create a net negative charge inside the cell.
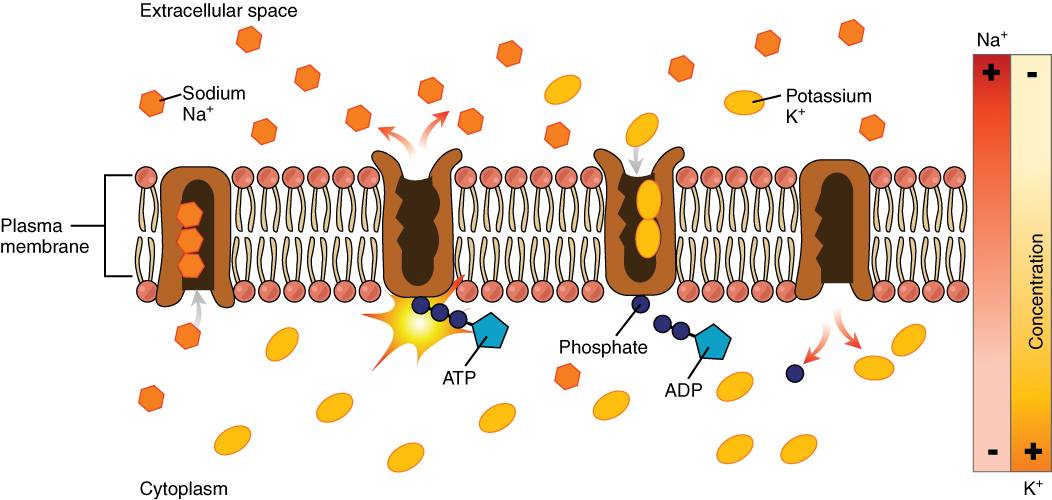
Action potential
When a stimulus reaches the neuron, it changes the electrochemical gradient, and the neuron sends a signal called an action potential. The stimulus opens sodium channels imbedded in the axon, thus diffusing sodium into the cell, making the net charge inside the cell positive. An action potential is said to be self-propagating, because if a stimulus is strong enough, the resulting action potential from the open sodium channels will be able to open its neighbor sodium channels as well, creating a chain reaction. However, this positive charge inside the cell is not maintained forever, because when the action potential has passed, the sodium channels close again, and potassium channels open. This diffuses potassium out of the cell and reactivates the sodium potassium pumps. The sodium potassium pumps then start to exchange two potassium ions into the cell for three sodium ions out of the cell, thus restoring the electrochemical gradient. There is nothing as a strong or weak action potential, because all the action potentials sent have the same strength. However, the frequency of action potentials can vary, thus creating a stronger sensation. If a stimulus is weaker than a set barrier, the neuron will not fire, and no action potential is sent. If a neuron is myelinated, the signal can skip from one node of Ranvier to the next one. This is because the thick sheath of myelin insulates the neuron, thus preventing loss of charge. The signal jumping from node to node means that the signal is faster, and that is requires less energy to restore balance after the action potential.
Synaptic communication
Neurons communicate with each other through synaptic gaps between the presynaptic neuron and the post synaptic neuron. When the action potential in the presynaptic neuron reaches the axon terminal, calcium channels open and diffuses calcium into the terminal buttons. This releases vesicles filled with neurotransmitters that diffuses through the synaptic gap, and are received by receptors on the post synaptic neuron. The binding of neurotransmitters to the receptors opens the sodium channels of the post synaptic neuron, creating a self-propagating action potential traveling along the axon. The neurotransmitter is then degraded and diffuses back to the presynaptic neuron to be reassembled. This process goes down the chain of neurons until it reaches the target tissue.
The hormone system and regulation of blood glucose levels
Homeostasis is the process of restoring balance in the body so it can function optimally, and it is controlled by the central nervous system and the endocrine system. The endocrine system uses feedback loops regulated by hormones to maintain homeostasis. One example of this is the regulation of blood sugar by insulin and glucagon. When the body detects a blood sugar level higher than a set threshold, it tells the pancreas to start producing insulin. Insulin is a hormone that has two primary function, to lower the blood sugar level, and to increase production of glycogen. Insulin lowers the blood sugar level by opening up protein channels, thus allowing glucose to diffuse into cells by facilitated diffusion. Additionally, insulin stimulates the liver to convert glucose into the polysaccharide glycogen, which is stored as granules in the hepatic cells of the liver, and in muscle cells. Both processes reduce the blood glucose levels. The opposite process happens when blood sugar levels are too low. When the body senses a low blood sugar, the pancreas starts to release glucagon instead of insulin. Glucagon stimulates the hydrolysis of the polysaccharide glycogen into the monosaccharide glucose, thus increasing blood sugar levels and maintaining homeostasis.
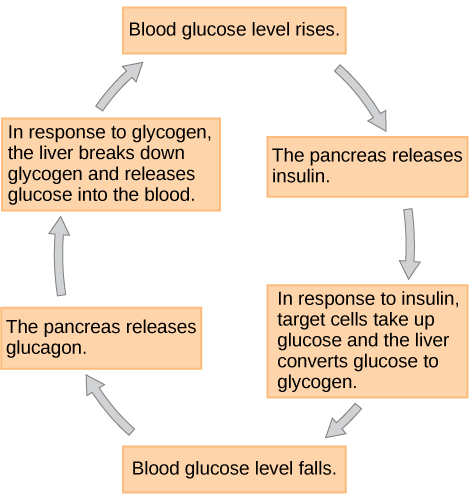
Menstrual cycle
Another example of a hormone regulated process is the menstrual cycle. The point of the menstrual cycle is to prepare an ovum (egg cell) in case of fertilization, and then release the ovum if it is not fertilized. In the start of the menstrual cycle, a hormone called GnRH (gonadotropin releasing hormone) is produced in the hypothalamus, and transported to the anterior pituitary gland, telling it to start producing two other hormones called LH (luteinizing hormone) and FSH (follicle stimulating hormone). The two hormones are transported to the ovaries, where they serve a series of functions. The first one is to stimulate the follicle cells in the ovaries to start producing estrogen. Estrogen increases the density of the blood vessels in the uterus to prepare for an ovum, in addition to stimulating the pituitary gland to produce even more LH and FSH. This is called a positive feedback loop. When levels of LH and FSH increases, the ovaries start to produce a structure called a graafian follicle, which is comprised of an oocyte and follicle cells. The oocyte is released in ovulation after levels of LH and FSH has spiked, but the outer ring of the graafian follicle remains in the ovaries and becomes a corpus luteum. The corpus luteum starts to produce a hormone called progesterone that maintains the vascular endometrium of the uterus, in addition to restricting further production of GnRH. This is called a negative feedback loop. Furthermore, the levels of LH and FSH stagnates due to no further production, which maintains the estrogen levels and prevents another graafian follicle from forming. After a few days, the corpus luteum disintegrates, and the drop in progesterone and estrogen means that the vascular endometrium can no longer be maintained, and that the hypothalamus should start to produce GnRH again, thus starting the menstrual cycle over again.
Helpful videos and further reading:
The digestive system: https://www.youtube.com/watch?v=Og5xAdC8EUI
The blood system: https://www.youtube.com/watch?v=NJzJKvkWWDc
The immune system: https://www.youtube.com/watch?v=z3M0vU3Dv8E
The respiratory system: https://www.youtube.com/watch?v=MrDbiKQOtlU
Blood glucose regulation: https://www.youtube.com/watch?v=Id2E72P8Fe0
Menstrual cycle: https://www.youtube.com/watch?v=tOluxtc3Cpw
The part about this chapter that fascinated me the most, was how a lot of processes in our body is a result of physical and chemical phenomena, such as how blood flows in the heart as a result of pressure differences, and how inhalation occurs as a result of a partial vacuum. I experienced this chapter as an incredibly interesting, but challenging chapter. Similar terms such as glucagon, glycogen, and glucose sometimes got me a little confused. However, I honestly found the menstrual cycle the most difficult, maybe because of all the different hormones, or because of the fact that I am a male.