This was a surprisingly hard topic, and probably the hardest topic so far. The topic goes all the way back to topic 2 and reintroduces metabolism, cellular respiration, and photosynthesis, but with much more depth. The metabolism section was pretty comprehensible, but the cellular respiration and photosynthesis section was just crazy. I felt like there was too much information conveyed over too short a span, so a lot of in-depth explanation was lost. I will try my best to clear up the confusing concepts and hopefully make it more understandable. I think Youtube videos are probably going to be life savers in this topic. The series Khan academy has on cellular respiration and photosynthesis is fantastic. I would also recommend the video explaining redox reactions from a biological perspective. The videos are linked at the bottom of the page.
Points for revision:
- Metabolic pathways, enzymes, and inhibition
- Electron transport chain and chemiosmosis
Metabolic pathways, enzymes, and inhibition
A metabolic pathway is a chain of enzyme catalyzed reactions, where substrate A is catalyzed into substrate B, which is catalyzed to substrate C and so on until it gets to the final product. A metabolic reaction can either by anabolic or catabolic. Anabolic reactions build up more complex molecules from less complex molecules and are endergonic. Endergonic reactions produce products with a higher energy than the reactants. One example of an endergonic reaction is photosynthesis. Catabolic reactions are the opposite of anabolic reactions. They break down more complex molecules into less complex molecules and are exergonic. Exergonic reactions produce a product with less energy than the reactants. One example of an exergonic reaction is cellular respiration. The sum of anabolic and catabolic reactions is the metabolism.
As mentioned, metabolic pathways are catalyzed by enzymes. Enzymes work by lowering the activation energy needed to make a reaction happen. They do this by the induced fit model. The induced fit model explains that an enzyme has an active site which can bind to a substrate. The active site and the substrate must be compatible in shape and charge, but when the substrate is bonded to an enzyme, the enzyme may change its shape to accommodate the substrate. Enzyme catalyzed reactions starts by a substrate bonding to an enzyme, changing the shape of the enzyme thus creating an enzyme substrate complex. The enzyme then reduces the substrate’s activation energy and transforms the substrate, which is then released as the product of the reaction. The enzyme can now go on and bind with the next substrate.
A metabolic pathway may want to be inhibited under some circumstances to avoid overproduction. Three ways of inhibition are discussed. The first way to inhibit enzyme catalyzed reactions is competitive inhibition. An inhibitor resembling the substrate permanently bonds with the enzyme, taking the place of the substrate. This reduced reactivity until more enzymes or substrate is added. The second method of inhibition is non-competitive inhibition. Here inhibitors bind to enzymes in another location than the active site called the allosteric site. The binding to the allosteric site changes the composition of the enzyme, which changes the shape of the active site. The targeted substrate thus cannot bind with the enzyme anymore. In non-competitive inhibition, in does not help to increase substrate concentrations as they are not competing. The only way to increase reactivity is to increase enzyme concentration. The last inhibition method is end-product inhibition. In end product inhibition the final product produced from the metabolic pathway inhibits the first step of the metabolic pathway, which shuts down the whole pathway. This reduces the yield of product, and when less product is produced, the reactivity in the first step increases. This prevents too much product from being produced. This is a good example of a negative feedback loop.
.png)
Respiration abstract
Respiration is basically the process of turning glucose into usable energy in the form of ATP. However, most of the ATP is not made until very late in the process. Respiration happens in four distinct steps. Glycolysis, the link reaction, the Krebs cycle, and the electron transport chain. Glycolysis and the link reaction are basically the preparation phases for the Krebs cycle, and the Krebs cycle is the preparation phase for the electron transport chain. Almost all of the ATP is produced in the electron transport chain by chemiosmosis. There are 4 essential molecules to understand when it comes to cellular respiration. NAD+, NADH, FAD, and FADH2. These are basically the electron carriers of the reaction, but what does that mean? Basically, many of the processes in cellular respiration are carried out by redox reactions. Redox reactions always happen in pairs, one oxidation and one reduction. I will explain this more clearly further down the page, and I would recommend reading that before continuing. For example, every time a molecule is decarboxylated (loses a carboxyl group COOH), it is an oxidation reaction because the carboxyl group loses a hydrogen and is turned into carbon dioxide. Since an oxidation reaction has occurred, a reduction has to follow. The NAD+ thus receives two electrons and a H+ ion (proton) and is reduced into NADH. The same happens with FAD, it is reduced to FADH2. Electrons are almost like an energy carrier, and since NADH and FADH2 are now carrying the electrons, they are also high in energy. NADH and FADH2 then goes through the electron transport chain, releasing more and more energy, and the released energy is used to make ATP.
Redox reactions
Redox reactions are central in both cellular respiration and photosynthesis, and it takes some chemistry knowledge to be able to fully understand it. Fortunately, no deep understanding is needed, as long as you understand what is happening. A redox reaction is comprised of two separate but intertwined reactions, an oxidation reaction, and a reduction reaction. A redox reaction is basically a transfer of electrons, from one substance to another. If an electron is given away, another substance has to take that electron. The two reactions therefore happen in pairs. An oxidation is a loss of electrons, while a reduction is a gain of electron. The mnemonic OIL RIG is commonly used. Is stands for Oxidation is loss, Reduction is gain. I usually think about reduction as reducing the charge, since electrons has a negative charge. In biology an oxidation reaction is associated with a gain of oxygen and loss of hydrogen, while a reduction reaction is associated with a loss of oxygen and gain of hydrogen. The name oxidation comes from the fact that gaining oxygen is associated with oxidation reactions. The reason why it is consisted with losing an electron is because oxygen has a high electron negativity, so it has a strong pull on the shared electrons in a covalent bond. This means that the oxygen is essentially removing an electron from the substance. In a reduction reaction, an electron is essentially gained since hydrogen has a low electron negativity, so hydrogen’s electron is pulled into the substance.
Glycolysis
Glycolysis is the first step of photosynthesis and it is divided into the investment phase and the payout phase. The goal of Glycolysis is to split up a six-carbon sugar into two three-carbon sugars. Glycolysis happens in the cytosol, and no organelles or oxygen is needed. In reality glycolysis is comprised of 10 steps, but a simplification is sufficient since it is the end products that are important. In the investment phase, two ATP are invested and turned into ADP. The two lost phosphate groups are attached to the six-carbon sugar to reduce its stability, this is called phosphorylation. The six carbon is now called a fructose-1,6-biphosphate. The phosphate is attached to carbon 1 and 6, and there are two phosphates, hence the name. The next step is called lysis (splitting), and the fructose-1,6-biphosphate is split into two 3-carbon molecules called glyeraldehyde-3-phosphate also known as G3P (phosphate attached at carbon number 3). The two G3P then goes on into an oxidation phase, which is followed by a reduction of NAD+ into NADH (it is not important to know how it is oxidized, the important part is that an oxidation has occurred, and that the reduction produced NADH). The resultant energy from the process is used to add a phosphate group to the G3P. Each of the two G3Ps have two phosphates, which means that they have 4 phosphates in total. The 4 phosphates in the final step are removed from the G3P and added to 4 ADP molecules by enzymes, making 4 ATP molecules. This is called the payout phase since an investment of 2 ATP has resulted in 4 ATP, making a net yield of 2 ATP. However, it is the three-carbon molecule without any phosphate groups called pyruvate, which is the true product of glycolysis. It is pyruvate that goes on into the link reaction.
In summary, a six-carbon sugar is split into two three-carbon molecules, which each produces one NADH, two ATP, and a pyruvate. Since two ATP are invested in the investment phase, glycolysis results in a gain of 2 NADH, 2 Pyruvate, and 2 ATP.

The link reaction
The link reaction processes the 2 pyruvate molecules into the final product acetyl CoA (acetyl coenzyme A), which enters into the Krebs cycle. That is why I call glycolysis and the link reaction the preparation phases for the Krebs cycle. The link reaction requires oxygen, and it occurs in the inner membrane of the mitochondria. In the link reaction, the two pyruvate molecules are decarboxylated thus producing 2 carbon dioxides (oxidation), and then bonded with coenzyme A. The reduction reaction following the oxidation reduces two NAD+ to NADH, and the bonded coenzyme makes 2 acetyl CoA molecules. The 2 acetyl CoA molecules then go into the Krebs cycle.
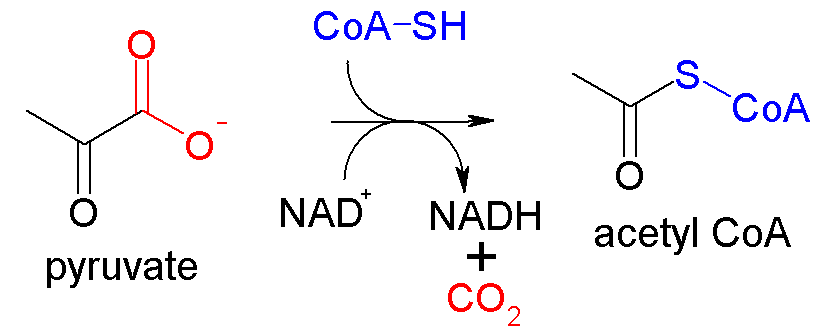
The Krebs cycle
The Krebs cycle occurs in the matrix of the mitochondrion, and even though it does not produce a lot of ATP, it is preparing for generating ATP in the electron transport chain by producing NADH and FADH2. It is called a cycle since the reactant is also the end product. The first step of the Krebs cycle is the addition of a 4-carbon molecule called oxaloacetate which binds with the 2-carbon acetyl CoA to form a 6-carbon molecule called citrate. The coenzyme A is lost in the process. The 6-carbon molecule then goes through 2 decarboxylation’s and two oxidations. As a rule of thumb, every time a decarboxylation occurs, CO2 is produced, and every time an oxidation occurs, either NADH or FADH2 is produced. In this case two NADH molecules are produced per citrate. Finally, the 4-carbon molecule goes through a series of oxidations and reductions, producing 1 ATP, 1 FADH2, and 1 NADH. The final 4-carbon molecule is oxaloacetate, which starts the cycle over again.
In summary, the Krebs cycle has produced 3 NADH, 1 FADH2, 1 ATP, and 2 CO2 per acetyl CoA. Since we had 2 acetyl CoA from the link reaction, the Krebs cycle produces 6 NADH, 2 FADH2, 2ATP, and 4 CO2 per glucose molecule.
Electron transport chain and chemiosmosis
The final step of respiration is the electron transport chain, and it is in this step where the bulk of ATP is produced. I would go back to topic 1 and review mitochondria structure before carrying on.
So far, we have 2 net ATP from glycolysis, and 2 ATP from the Krebs cycle. However, we also have 4 NADH from glycolysis and the link reaction, and 6 NADH and 2 FADH2 from the Krebs cycle. It is these electron carrier molecules that will produce the ATP by chemiosmosis. Chemiosmosis is almost like osmosis, but instead of water moving through a semipermeable membrane, it is ions. The electron transport chain happens in the inner mitochondrial membrane and the cristae membrane. The cristae membrane has embedded molecules that have very different electron negativities. So, when NADH or FADH2 enters the membrane, its electrons are pulled off by one of these molecules, which then loses the electrons to a molecule with even higher electron negativity. Each time a electron is transferred down the chain, some energy is lost. At the end of the reaction, the electrons are bonded to the highly electron negative oxygen, which makes water. This is the first step where oxygen is absolutely necessary, and is why we need to breath in oxygen, and why we breath out water. It is also the reason why aerobic respiration produces so much more ATP. Without oxygen being there to carry out the electron, the electron transport chain would function.
The energy released by electrons going down the electron transport chain is used to power membrane pumps in the cristae membrane that pumps hydrogen ions out of the inner membrane into the inner membrane space. These hydrogen ions want to go back to the mitochondrial matrix since the concentration is less there, but they cannot pass through the membrane. However, there is an imbedded protein in the inner membrane called ATP synthase that allows hydrogen ions to flow in. ATP synthase works like a hydro power plant. The flow of hydrogen ions flowing through the ATP synthase spins a turbine, which gives the energy to add a phosphate group to ADP, turning it in to ATP. The process of the electron transport chain and chemiosmosis is called oxidative phosphorylation. Since NADH enters at a higher energy level than FADH2, it can theoretically produce 3 ATP, and FADH2 can produce 2 ATP. The real number is actually much smaller.
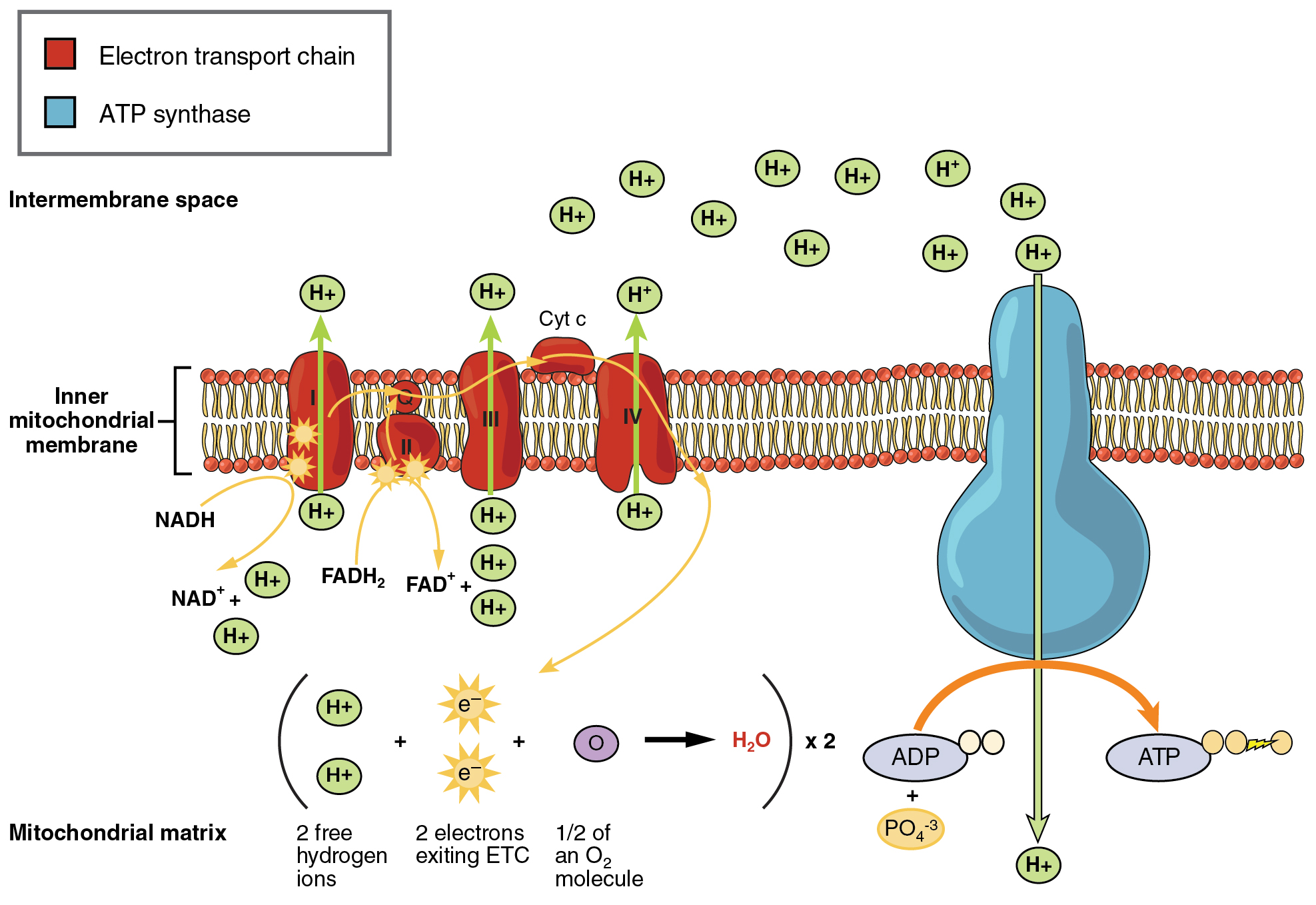
So, to summarize the ATP yield by aerobic respiration. 2 ATP and 2 NADH are produced by glycolysis. 2 Additional NADH are produced by the link reaction. Lastly, the Krebs cycle produces 2ATP, 6 NADH, and 2 FADH2. This gives a total of 4 ATP, 10 NADH, and 2 FADH2. This gives a total yield of 38 ATP molecules. The realistic yield is more around 30-32 ATP since no cells are this effective.
The reaction for aerobic respiration is commonly denoted as C6H12O6 + 6O2 → 6CO2 + 6H2O + ATP. Now we know that glucose is needed to start the reaction of glycolysis, oxygen is needed to carry on the electron transport chain, CO2 is produced by decarboxylation, and water is produced by the electron transport chain. We also know how the ATP is produced, which sums up aerobic respiration.
Photosynthesis abstract
Photosynthesis is the reaction that turns inorganic carbon compound into organic compounds, usually glucose. It is usually said to be the opposite reaction of respiration, and although it is true, they both have a lot of similarities. They both involve an electron transport chain that produces ATP, and they both have cycle of some kind. In photosynthesis the cycle is called the Calvin cycle. Photosynthesis is divided into two processes. The light dependent, and the light independent. Both reactions occur simultaneously, but only the light dependent reaction needs photon energy from light. The light dependent reactions prepare material and energy for the light independent reaction, which turns inorganic carbon dioxide into organic compounds with the energy provided from the light dependent reaction. Unlike respiration, the process starts in the thylakoids before moving out to the stroma, whereas respirations start in the cytoplasm, and then moves into the inner membrane of mitochondria. I would recommend going back to topic 1 and revise chloroplast structure, since all the reactions in photosynthesis occurs in the chloroplast.
Light dependent reaction
The light dependent reaction is similar to the electron transport chain in respiration in that it also includes an electron transport chain. However, instead of needing a molecule like NADH to provide energy, the energy in the light dependent reactions comes from photons. In the thylakoid membrane there are embedded structures called photosystems. In plants there are 2 photosystems, Photosystem II(P680) and photosystem I(P700). Photosystem II comes in before photosystem I, it was just discovered after. The numbering stands for the wavelength it absorbs the best (680nm and 700nm). Each photosystem has pigments, namely chlorophyll and carotenoids, and a reaction center. The reaction centers have a primary electron acceptor which receives the electron which gets excited by a photon and goes down the transport chain.
When sunlight hits photosystem II, some of its energy is absorbed by the pigments in the photosystem, which excites an electron which is received by the primary acceptor in the reaction center. To replace the electron in the pigment which got transferred to the primary acceptor, water is split by an enzyme in a process called photolysis. The resultant products are electrons and oxygen. The electrons are used to replace the excited electron, and oxygen is released as a biproduct. The electron that got excited in the primary acceptor is passed down an electron transport chain by electron carriers, releasing energy which is used to pump hydrogen ions into the thylakoid space by cytochrome complexes. Chemiosmosis then happens in the same fashion as in respiration, where the hydrogen ions move past an ATP synthase which generates the energy needed to turn ADP into ATP. ATP is the final product of the first part of the light dependent reaction.
The second part of the light dependent reaction happens in photosystem I. The pigments in photosystem I absorbs energy from a photon, and an electron is excited the same way as in photosystem II. In this process no water is split because the excited electron is just replaced by the deenergized electron from photosystem II. The excited electron then moves down another transport chain by electron carriers, and in the end, it is used to reduce NADP+ into NADPH by a reaction catalyzed by an enzyme called NADP reductase.
To sum it up, the final products of the light dependent reactions are ATP and NADPH, which are the necessary materials for the light independent reaction.
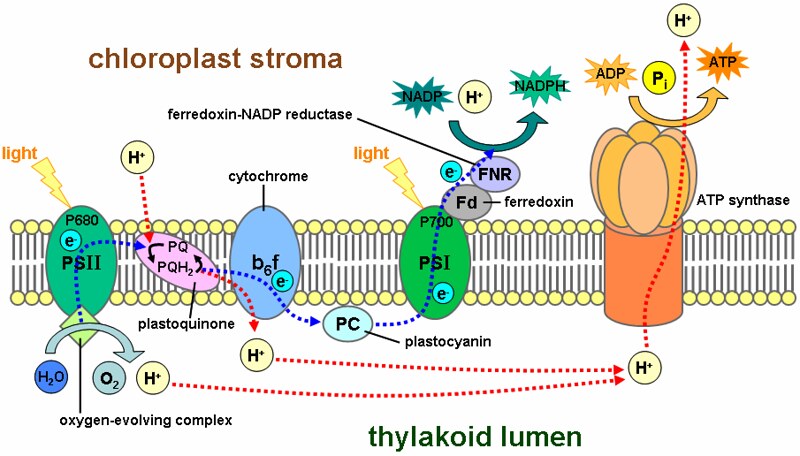
Light independent reaction
The light independent reaction is mainly comprised by the Calvin cycle, which is the process that can produce organic glucose. In the Calvin cycle, a 5-carbon compound called ribulose biphosphate (RuBP) is bonded to an incoming CO2 molecule by enzymes in a reaction called carbon fixation. This produces an unstable 6-carbon molecule which is broken up into two 3-carbon molecules called glycerate-3-phosphate (GP). The products from the light dependent reactions, APT and NADPH is then used to process the GP into a new 3-carbon compound called triose phosphate. This is done by oxidizing NADPH into NADP+, and the following reduction reaction reduces the GP into triose phosphate. The energy needed is provided by ATP. 12 triose phosphate molecules are made, but only 2 of the molecules are used to create a 6-carbon carbohydrate. The rest of the triose phosphate is recycled back to RuBP to start the cycle again. The energy needed for this is provided by the rest of the ATP produced from the light dependent reactions.
To summarize, photosynthesis is written as 6CO2+ 12H2O → C6H12O6 + 6H2O + 6O2. Now we know that CO2 is needed to start the Calvin cycle, water is needed to replace the excited electron in photosystem II, oxygen is produced as a biproduct of the reaction, and a carbohydrate is the final product of the Calvin cycle.
Helpful videos:
Redox reactions: https://www.youtube.com/watch?v=_KyyVhlUDNU
Khan academy respiration series: https://www.youtube.com/playlist?list=PLSQl0a2vh4HCKeX3g-Mj5wXS0nfDeDSGS
Khan academy photosynthesis series: https://www.youtube.com/playlist?list=PLAE36CEFE9200FDDD
This topic was honestly very hard, and I would probably have gotten a headache if it was not for Khan academy. However, I feel very satisfied now that I have mastered the material. The diagrams in the book were very helpful as everything is much more simplified than those on the internet. However, it was much easier to understand the topic with the help of videos and animation. As you may have noticed, the page turned out to be really long. I think that reflects the depth of this topic.